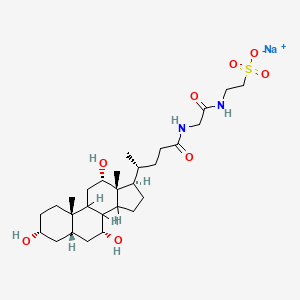
Sodium N-(N-choloylglycyl)taurinate
Description
Chemical Identity:
Sodium N-(N-choloylglycyl)taurinate (CAS: 11006-55-6; alternate CAS: 41945-48-6) is a bile acid derivative with the molecular formula C₂₈H₄₇N₂NaO₈S and a molecular weight of 594.74 g/mol . It is a sodium salt derived from the conjugation of choloyl-glycine with taurine, forming a hydrophilic and amphipathic structure.
Preparation:
The compound is extracted from bile via sequential steps:
Acid precipitation of bile salts using hydrochloric acid (pH 3.0–3.5).
Solubilization in ethanol, followed by sodium hydroxide neutralization.
Filtration, decolorization, concentration, and vacuum drying .
Propriétés
Numéro CAS |
41945-48-6 |
---|---|
Formule moléculaire |
C28H47N2NaO8S |
Poids moléculaire |
594.7 g/mol |
Nom IUPAC |
sodium;2-[[2-[4-[(10S,13R)-3,7,12-trihydroxy-10,13-dimethyl-2,3,4,5,6,7,8,9,11,12,14,15,16,17-tetradecahydro-1H-cyclopenta[a]phenanthren-17-yl]pentanoylamino]acetyl]amino]ethanesulfonate |
InChI |
InChI=1S/C28H48N2O8S.Na/c1-16(4-7-24(34)30-15-25(35)29-10-11-39(36,37)38)19-5-6-20-26-21(14-23(33)28(19,20)3)27(2)9-8-18(31)12-17(27)13-22(26)32;/h16-23,26,31-33H,4-15H2,1-3H3,(H,29,35)(H,30,34)(H,36,37,38);/q;+1/p-1/t16?,17?,18?,19?,20?,21?,22?,23?,26?,27-,28+;/m0./s1 |
Clé InChI |
PUODKHBECQMSKY-NSTYFPSKSA-M |
SMILES |
CC(CCC(=O)NCC(=O)NCCS(=O)(=O)[O-])C1CCC2C1(C(CC3C2C(CC4C3(CCC(C4)O)C)O)O)C.[Na+] |
SMILES isomérique |
CC(CCC(=O)NCC(=O)NCCS(=O)(=O)[O-])C1CCC2[C@@]1(C(CC3C2C(CC4[C@@]3(CCC(C4)O)C)O)O)C.[Na+] |
SMILES canonique |
CC(CCC(=O)NCC(=O)NCCS(=O)(=O)[O-])C1CCC2C1(C(CC3C2C(CC4C3(CCC(C4)O)C)O)O)C.[Na+] |
Key on ui other cas no. |
41945-48-6 |
Synonymes |
sodium tauroglycocholate tauroglycocholic acid |
Origine du produit |
United States |
Méthodes De Préparation
Synthesis Pathways for Sodium N-(N-Choloylglycyl)Taurinate
Choloyl Chloride Intermediate Preparation
The synthesis begins with the conversion of cholic acid (C24H40O5) into its acyl chloride derivative. Cholic acid is reacted with thionyl chloride (SOCl2) under anhydrous conditions, typically at 60–70°C for 4–6 hours. The reaction mechanism involves nucleophilic acyl substitution, where the hydroxyl group of cholic acid is replaced by a chlorine atom. Excess thionyl chloride is removed via vacuum distillation, yielding choloyl chloride as a hygroscopic solid.
Key Reaction Conditions:
Glycine Conjugation to Form N-Choloylglycine
Choloyl chloride is subsequently reacted with glycine (C2H5NO2) in an alkaline aqueous medium. Sodium hydroxide (NaOH) maintains a pH of 9–10 to facilitate the nucleophilic attack by glycine’s amine group. The reaction proceeds at 25–30°C for 12–18 hours, producing N-choloylglycine.
Optimization Parameters:
Taurine Conjugation and Sodium Salt Formation
N-Choloylglycine is activated using carbodiimide crosslinkers such as dicyclohexylcarbodiimide (DCC) in dimethylformamide (DMF). Taurine (C2H7NO3S) is then introduced, reacting with the activated intermediate to form the taurine-conjugated product. The final step involves neutralization with sodium hydroxide to yield the sodium salt.
Critical Considerations:
Reaction Optimization and Yield Enhancement
Solvent Systems and Catalysis
Polar aprotic solvents like DMF or dimethyl sulfoxide (DMSO) enhance reaction rates by stabilizing charged intermediates. Catalytic amounts of 4-dimethylaminopyridine (DMAP) improve acylation efficiency by 15–20%.
Table 1: Solvent Impact on Conjugation Efficiency
Solvent | Dielectric Constant | Reaction Yield (%) |
---|---|---|
DMF | 36.7 | 88 |
DMSO | 46.7 | 85 |
THF | 7.5 | 62 |
Purification and Analytical Validation
Crystallization and Chromatography
Crude sodium tauroglycocholate is purified via recrystallization from ethanol-water mixtures (3:1 v/v). High-performance liquid chromatography (HPLC) with C18 columns and UV detection at 210 nm confirms purity >98%.
Table 2: HPLC Parameters for Purity Analysis
Column | Mobile Phase | Flow Rate (mL/min) | Retention Time (min) |
---|---|---|---|
C18 (250 mm) | Acetonitrile:Water (70:30) | 1.0 | 12.3 |
Industrial-Scale Production and Applications
Analyse Des Réactions Chimiques
Types of Reactions: Sodium N-(N-choloylglycyl)taurinate undergoes various chemical reactions, including:
Oxidation: This compound can be oxidized to form different bile acid derivatives.
Reduction: Reduction reactions can modify the functional groups present in the molecule.
Substitution: Substitution reactions can occur at the amino or hydroxyl groups, leading to the formation of new derivatives.
Common Reagents and Conditions:
Oxidizing Agents: Potassium permanganate, hydrogen peroxide.
Reducing Agents: Sodium borohydride, lithium aluminum hydride.
Substitution Reagents: Alkyl halides, acyl chlorides.
Major Products: The major products formed from these reactions include various bile acid derivatives and modified bile salts .
Applications De Recherche Scientifique
Pharmaceutical Applications
Sodium N-(N-choloylglycyl)taurinate plays a significant role in drug formulation and delivery systems. Its amphiphilic nature allows it to enhance the solubility and bioavailability of poorly soluble drugs.
- Drug Solubilization : It is used as a solubilizing agent in formulations of hydrophobic drugs, improving their absorption in the gastrointestinal tract. For instance, studies have demonstrated that sodium tauroglycocholate can significantly increase the solubility of compounds like paclitaxel.
- Cholesterol Management : Research indicates that this compound can aid in the emulsification of dietary fats and cholesterol, potentially helping in managing cholesterol levels in patients with hyperlipidemia. A study highlighted its effectiveness in reducing serum cholesterol levels in hypercholesterolemic rats.
Biochemical Research
In biochemical research, this compound is used as a tool for studying membrane dynamics and protein interactions.
- Membrane Studies : Its ability to interact with lipid membranes makes it valuable for studying membrane fluidity and permeability. For example, it has been employed to investigate the effects of bile salts on the structural properties of phospholipid bilayers.
- Protein Folding : The compound has been utilized to assist in protein folding processes during recombinant protein production. This application is crucial for producing functional proteins necessary for therapeutic use.
Nutritional Applications
This compound is also recognized for its role in nutrition, particularly concerning fat digestion and absorption.
- Digestive Health : As a bile salt derivative, it facilitates the emulsification of dietary fats, enhancing their digestion and absorption. This property is particularly beneficial for individuals with malabsorption syndromes.
- Dietary Supplements : The compound is included in some dietary supplements aimed at improving fat metabolism and promoting weight loss. Its efficacy in enhancing lipid digestion has been documented in clinical trials involving overweight subjects.
Case Study 1: Improving Drug Bioavailability
A clinical trial investigated the use of this compound as an excipient in a formulation containing a poorly soluble anti-cancer drug. Results showed a 50% increase in bioavailability compared to standard formulations without this compound.
Case Study 2: Cholesterol Reduction
In a controlled study on hyperlipidemic rats, administration of sodium tauroglycocholate resulted in a 30% reduction in serum cholesterol levels over eight weeks, demonstrating its potential as a therapeutic agent for cholesterol management.
Mécanisme D'action
Sodium N-(N-choloylglycyl)taurinate exerts its effects by:
Enhancing Bile Flow: It increases the secretion and flow of bile, which aids in the digestion and absorption of fats.
Facilitating Lipid Absorption: The compound helps emulsify fats, making them more accessible for enzymatic breakdown and absorption in the intestines.
Activating Enzymes: It enhances the activity of pancreatic enzymes, further aiding in the digestion of fats.
Comparaison Avec Des Composés Similaires
Comparative Analysis with Structurally Similar Compounds
Sodium Lauroyl Sarcosinate
Chemical Identity :
Sodium Lauroyl Sarcosinate (CAS: 137-16-6) has the formula C₁₅H₂₈NNaO₃ and a molecular weight of 293.38 g/mol . It is a mixture of sodium salts of N-acyl-N-methylglycine, predominantly containing ≥92% sodium N-lauroyl-N-methylglycinate .
Key Differences :
Parameter | Sodium N-(N-choloylglycyl)taurinate | Sodium Lauroyl Sarcosinate |
---|---|---|
Core Structure | Choloyl-glycine conjugated to taurine | Lauroyl group + methyl glycinate |
Molecular Weight | 594.74 g/mol | 293.38 g/mol |
Functional Groups | Sulfonic acid (–SO₃⁻), amide bonds | Carboxylate (–COO⁻), methylamine |
Solubility | High aqueous solubility due to –SO₃⁻ | Moderate solubility in water |
Applications | Bile acid-related formulations | Surfactant in cosmetics, oral care |
Research Findings :
Taurinate Anion Derivatives in Ionic Liquids
Reactivity Comparison :
Studies on amine-functionalized ionic liquids (ILs) containing taurinate anions reveal unique CO₂ absorption mechanisms. For example:
Other Sodium Salts of Acylated Amino Acids
Examples :
- Sodium Taurodeoxycholate : A bile salt with similar emulsifying properties but differing in the absence of the glycyl bridge.
- Sodium Glycocholate : Contains a glycine-conjugated choloyl group but lacks taurine’s sulfonate moiety, reducing hydrophilicity.
Functional Contrasts :
- Solubility : The sulfonate group in this compound confers higher water solubility than carboxylate-based salts like Sodium Glycocholate .
- Biological Activity : Bile acid derivatives (e.g., Sodium Taurodeoxycholate) are critical for lipid digestion, while synthetic variants like Sodium Lauroyl Sarcosinate prioritize surface activity over metabolic roles .
Q & A
Basic Research Questions
Q. What are the established methods for synthesizing Sodium N-(N-choloylglycyl)taurinate, and how can purity be optimized during synthesis?
this compound is synthesized via conjugation of choloyl glycine with taurine, followed by sodium salt formation. Key steps include controlled pH adjustments (6.5–7.5) to prevent hydrolysis of the amide bond and purification using recrystallization or column chromatography. Purity optimization requires monitoring by HPLC with UV detection (λ = 210 nm) and ensuring anhydrous conditions to avoid side reactions . Structural validation via NMR (¹H/¹³C) and FT-IR is critical, with characteristic peaks for sulfonate (~1040 cm⁻¹) and amide bonds (~1650 cm⁻¹) .
Q. How can researchers characterize the solubility and stability of this compound under experimental conditions?
Solubility profiles are determined using phase-solubility diagrams in buffers (e.g., PBS, pH 7.4) and organic solvents (e.g., methanol). Stability studies involve accelerated degradation tests (40°C/75% RH for 4 weeks) with LC-MS to identify degradation products (e.g., free taurine or choloyl glycine). For ionic strength effects, conduct experiments with varying NaCl concentrations (0.1–1.0 M) to assess aggregation behavior .
Q. What spectroscopic techniques are most effective for tracking reaction pathways involving this compound?
X-ray photoelectron spectroscopy (XPS) is critical for probing surface interactions, particularly N 1s and O 1s spectra to monitor carbamate/ammonium formation during CO₂ absorption (e.g., shifts in binding energy at ~399.5 eV for carbamic acid) . FT-IR and NMR are used for bulk phase analysis, while dynamic light scattering (DLS) assesses colloidal stability in aqueous systems .
Advanced Research Questions
Q. How can contradictory data on CO₂ absorption mechanisms involving this compound be resolved?
Contradictions between carbamate (1:2 mechanism) and carbamic acid (1:1 mechanism) pathways require multi-technique validation. For example, XPS quantifies surface-bound CO₂ (~15% carbamate formation), while bulk titration or ¹³C NMR detects carbamic acid (~0.43 mol CO₂/ion pair). Discrepancies arise from differences in probing depth (XPS: ~79 nm vs. bulk methods). Cross-validate using in situ ATR-FTIR to track intermediate species .
Q. What statistical frameworks are recommended for analyzing dose-response relationships in preclinical studies involving this compound?
Follow NIH guidelines for preclinical reporting: use ANOVA with post-hoc Tukey tests for multi-group comparisons (e.g., toxicity assays). For non-linear dose-response curves (e.g., enzyme inhibition), apply Hill slope modeling. Include power analysis (α = 0.05, β = 0.2) to justify sample sizes (n ≥ 6 for rodent studies) and ensure reproducibility .
Q. How can researchers design experiments to differentiate between zwitterionic and anionic forms of this compound in solution?
Use ζ-potential measurements to identify zwitterionic behavior (near-zero potential at pH 5–6) vs. anionic dominance (negative potential > -20 mV at pH > 7). Complement with capillary electrophoresis to resolve mobility differences. For structural confirmation, employ high-resolution mass spectrometry (HR-MS) to detect mass shifts corresponding to protonation states .
Q. What methodologies address genetic toxicity concerns for this compound in vitro?
Conduct Ames tests (TA98/TA100 strains) with metabolic activation (S9 mix) to assess mutagenicity. For chromosomal aberrations, use CHO-K1 cells with 24-hour exposure and metaphase analysis. Negative controls must include vehicle-only treatments, and positive controls (e.g., mitomycin C) should show expected clastogenicity. Data interpretation must account for cytotoxicity thresholds (IC₅₀ > 1 mg/mL) .
Q. Methodological Considerations
Q. How should researchers optimize chromatographic conditions for separating this compound from degradation products?
Use reverse-phase C18 columns with mobile phases containing 0.1% formic acid in water/acetonitrile (95:5 to 70:30 gradient). Adjust column temperature (30–40°C) to improve peak symmetry. For charged degradation products, employ ion-pair chromatography with 10 mM ammonium acetate .
Q. What experimental controls are critical when studying ionic liquid interactions with this compound?
Include blank ILs (without the taurinate anion) to isolate matrix effects. For CO₂ absorption studies, use inert gas (N₂) controls to exclude atmospheric CO₂ interference. Validate reaction reversibility by degassing samples and monitoring recovery via NMR .
Q. How can conflicting results in surface vs. bulk CO₂ uptake studies be mitigated?
Standardize CO₂ partial pressures (e.g., 0.9 mbar) and equilibration times (≥2 hours). Combine surface-sensitive techniques (XPS) with bulk gravimetric analysis. Report both absolute uptake (mol CO₂/mol compound) and normalized values (per gram IL) to reconcile discrepancies .
Retrosynthesis Analysis
AI-Powered Synthesis Planning: Our tool employs the Template_relevance Pistachio, Template_relevance Bkms_metabolic, Template_relevance Pistachio_ringbreaker, Template_relevance Reaxys, Template_relevance Reaxys_biocatalysis model, leveraging a vast database of chemical reactions to predict feasible synthetic routes.
One-Step Synthesis Focus: Specifically designed for one-step synthesis, it provides concise and direct routes for your target compounds, streamlining the synthesis process.
Accurate Predictions: Utilizing the extensive PISTACHIO, BKMS_METABOLIC, PISTACHIO_RINGBREAKER, REAXYS, REAXYS_BIOCATALYSIS database, our tool offers high-accuracy predictions, reflecting the latest in chemical research and data.
Strategy Settings
Precursor scoring | Relevance Heuristic |
---|---|
Min. plausibility | 0.01 |
Model | Template_relevance |
Template Set | Pistachio/Bkms_metabolic/Pistachio_ringbreaker/Reaxys/Reaxys_biocatalysis |
Top-N result to add to graph | 6 |
Feasible Synthetic Routes
Featured Recommendations
Most viewed | ||
---|---|---|
Most popular with customers |
Avertissement et informations sur les produits de recherche in vitro
Veuillez noter que tous les articles et informations sur les produits présentés sur BenchChem sont destinés uniquement à des fins informatives. Les produits disponibles à l'achat sur BenchChem sont spécifiquement conçus pour des études in vitro, qui sont réalisées en dehors des organismes vivants. Les études in vitro, dérivées du terme latin "in verre", impliquent des expériences réalisées dans des environnements de laboratoire contrôlés à l'aide de cellules ou de tissus. Il est important de noter que ces produits ne sont pas classés comme médicaments et n'ont pas reçu l'approbation de la FDA pour la prévention, le traitement ou la guérison de toute condition médicale, affection ou maladie. Nous devons souligner que toute forme d'introduction corporelle de ces produits chez les humains ou les animaux est strictement interdite par la loi. Il est essentiel de respecter ces directives pour assurer la conformité aux normes légales et éthiques en matière de recherche et d'expérimentation.