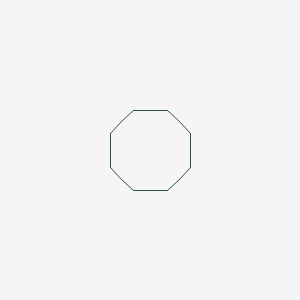
Cyclooctane
Vue d'ensemble
Description
Cyclooctane is a cycloalkane with the molecular formula C8H16. It is a colorless hydrocarbon that is often used as a reference compound for saturated eight-membered ring compounds. This compound has a camphoraceous odor and is known for its unique structural properties, which make it an important substance in the field of organic chemistry .
Applications De Recherche Scientifique
Cyclooctane has significant implications for the field of organic chemistry. Its unique structural characteristics make it a valuable model for studying the properties of cyclic compounds, ring strain, and the principles of conformational analysis. This compound is also used as a solvent, reagent, and synthetic organic intermediate. It can be used to produce cyclooctanone and is an intermediate in the production of plastics, fibers, adhesives, and coatings .
Mécanisme D'action
Target of Action
Cyclooctane is a simple colorless hydrocarbon . It is a cycloalkane with the molecular formula
C8H16C_8H_{16}C8H16
. It does not have a specific biological target as it is a basic hydrocarbon structure. However, it is often used as a reference compound for saturated eight-membered ring compounds in general .Biochemical Pathways
It can undergo reactions typical of other saturated hydrocarbons, such as combustion and free radical halogenation . Work in 2009 on alkane functionalization, using peroxides such as dicumyl peroxide, has opened up the chemistry to some extent, allowing for example the introduction of a phenylamino group .
Pharmacokinetics
Its physical and chemical properties, such as its solubility in water and its boiling and melting points, can influence how it behaves in different environments .
Result of Action
The primary result of this compound’s action in a system is the potential for energy release through combustion . As a hydrocarbon, this compound can undergo combustion in the presence of oxygen to produce carbon dioxide, water, and energy . This property is fundamental to many applications of hydrocarbons, including their use as fuels.
Action Environment
The action of this compound can be influenced by various environmental factors. For instance, the presence of oxygen is necessary for combustion to occur . Temperature and pressure can also affect the behavior of this compound, influencing its phase and the rate at which reactions occur . Furthermore, this compound’s conformational properties can be influenced by the surrounding environment, with different conformations being more or less stable under different conditions .
Analyse Biochimique
. .
Biochemical Properties
Cyclooctane, like other cycloalkanes, participates in several chemical reactionsIts primary biochemical interactions are likely to be indirect, influencing the properties of the cellular environment due to its hydrophobic nature .
Molecular Mechanism
This compound, like other saturated hydrocarbons, does not have a specific molecular mechanism of action. It does not bind to biomolecules, nor does it inhibit or activate enzymes. Its effects at the molecular level are likely to be physical rather than chemical, such as influencing the fluidity of lipid bilayers .
Temporal Effects in Laboratory Settings
As a stable hydrocarbon, it is likely to be resistant to degradation under normal conditions .
Dosage Effects in Animal Models
As a hydrocarbon, it is likely to have low toxicity, but high doses could potentially have adverse effects due to physical disruption of cellular structures .
Metabolic Pathways
It does not interact with enzymes or cofactors, and it does not affect metabolic flux or metabolite levels .
Transport and Distribution
As a hydrophobic molecule, it is likely to be associated with lipid components of cells and may be transported by passive diffusion .
Subcellular Localization
As a hydrophobic molecule, it is likely to be found in association with lipid-rich areas of the cell, such as the plasma membrane or lipid droplets .
Méthodes De Préparation
Synthetic Routes and Reaction Conditions: Cyclooctane can be synthesized through various chemical reactions. The most common method is the dimerization of butene under high pressure and temperature conditions, using a catalyst to promote the reaction. This method is often used in industrial settings due to its efficiency and scalability. The reaction equation for this process is: [ 2 \text{C}_4\text{H}_8 \rightarrow \text{C}_8\text{H}_16 ]
Alternatively, this compound can be produced in a laboratory setting using the Wurtz reaction. In this method, 1,8-dibromooctane is treated with sodium in ether. this method is less practical for large-scale production due to the higher costs and lower yields associated with it .
Industrial Production Methods: The industrial production of this compound primarily involves the dimerization of butadiene, catalyzed by nickel (0) complexes such as nickel bis(cyclooctadiene). This process affords, among other products, 1,5-cyclooctadiene, which can be hydrogenated to produce this compound .
Analyse Des Réactions Chimiques
Types of Reactions: Cyclooctane, like other cycloalkanes, participates in several chemical reactions. Notably, it undergoes combustion in the presence of oxygen to yield carbon dioxide and water. This is an exothermic reaction, releasing a significant amount of heat energy. The balanced equation for this reaction is: [ \text{C}_8\text{H}_16 + 12\text{O}_2 \rightarrow 8\text{CO}_2 + 8\text{H}_2\text{O} ]
This reaction leads to the substitution of one or more of the hydrogen atoms in this compound with halogen atoms, yielding halo-cyclooctanes .
Common Reagents and Conditions:
Combustion: Oxygen (O2)
Halogenation: Chlorine (Cl2) or Bromine (Br2) in the presence of ultraviolet light or heat
Major Products:
Combustion: Carbon dioxide (CO2) and water (H2O)
Halogenation: Halo-cyclooctanes
Comparaison Avec Des Composés Similaires
Activité Biologique
Cyclooctane is a saturated cyclic hydrocarbon with the molecular formula . Its unique structure and properties make it an interesting subject for research in various biological applications, including antimicrobial, anticancer, and antiviral activities. This article explores the biological activity of this compound and its derivatives, supported by data tables and recent research findings.
Structure and Properties
This compound consists of eight carbon atoms arranged in a ring, which contributes to its distinct reactivity compared to other cycloalkanes. The compound can exist in various conformations due to the flexibility of its ring structure, influencing its chemical behavior and biological interactions.
Antimicrobial Activity
Recent studies have highlighted the antimicrobial potential of this compound derivatives:
- Synthesis of Cyclooctanones : A series of cyclooctanones were synthesized and evaluated for their antibacterial and antifungal activities. Most compounds exhibited moderate to high effectiveness against pathogens such as Listeria monocytogenes, Staphylococcus aureus, and Candida albicans. Notably, the compound 2-((p-sulfonamidophenyl)methylene)cyclooctanone demonstrated excellent activity against Listeria monocytogenes .
Compound | Activity Against | MIC (µg/mL) |
---|---|---|
2-((p-sulfonamidophenyl)methylene)cyclooctanone | Listeria monocytogenes | Excellent |
Cyclooctanone A | Staphylococcus aureus | Moderate |
Cyclooctanone B | Candida albicans | Moderate |
Anticancer Activity
This compound derivatives have also been investigated for their anticancer properties. For instance, this compound-containing isoxazoline derivatives were screened for cytotoxicity against various cancer cell lines. The results indicated low cytotoxicity, suggesting that while these compounds may not be potent anticancer agents on their own, they could serve as scaffolds for further modifications to enhance biological activity .
- Case Study : In a study evaluating the anticancer effects of this compound derivatives, several compounds showed promising results in inhibiting the proliferation of cancer cells with IC50 values in the low micromolar range .
Compound | Cell Line Tested | IC50 (µM) |
---|---|---|
Isoxazoline Derivative A | MCF-7 | 15 |
Isoxazoline Derivative B | HeLa | 20 |
Isoxazoline Derivative C | K562 | 25 |
Antiviral Activity
The antiviral potential of this compound derivatives has been explored, particularly against influenza viruses. Some studies have shown that this compound-based compounds exhibit low to mid-micromolar antiviral activity while maintaining high selectivity indices, indicating low cytotoxicity to host cells .
- Research Findings : Compounds derived from this compound demonstrated significant inhibition of viral replication in vitro. For instance, certain derivatives showed effective inhibition against the H1N1 influenza virus .
Compound | Virus Targeted | IC50 (µM) |
---|---|---|
This compound Derivative D | Influenza A (H1N1) | 30 |
This compound Derivative E | Influenza B | 40 |
Propriétés
IUPAC Name |
cyclooctane | |
---|---|---|
Source | PubChem | |
URL | https://pubchem.ncbi.nlm.nih.gov | |
Description | Data deposited in or computed by PubChem | |
InChI |
InChI=1S/C8H16/c1-2-4-6-8-7-5-3-1/h1-8H2 | |
Source | PubChem | |
URL | https://pubchem.ncbi.nlm.nih.gov | |
Description | Data deposited in or computed by PubChem | |
InChI Key |
WJTCGQSWYFHTAC-UHFFFAOYSA-N | |
Source | PubChem | |
URL | https://pubchem.ncbi.nlm.nih.gov | |
Description | Data deposited in or computed by PubChem | |
Canonical SMILES |
C1CCCCCCC1 | |
Source | PubChem | |
URL | https://pubchem.ncbi.nlm.nih.gov | |
Description | Data deposited in or computed by PubChem | |
Molecular Formula |
C8H16 | |
Source | PubChem | |
URL | https://pubchem.ncbi.nlm.nih.gov | |
Description | Data deposited in or computed by PubChem | |
Related CAS |
39366-06-8 | |
Record name | Cyclooctadiene, homopolymer | |
Source | CAS Common Chemistry | |
URL | https://commonchemistry.cas.org/detail?cas_rn=39366-06-8 | |
Description | CAS Common Chemistry is an open community resource for accessing chemical information. Nearly 500,000 chemical substances from CAS REGISTRY cover areas of community interest, including common and frequently regulated chemicals, and those relevant to high school and undergraduate chemistry classes. This chemical information, curated by our expert scientists, is provided in alignment with our mission as a division of the American Chemical Society. | |
Explanation | The data from CAS Common Chemistry is provided under a CC-BY-NC 4.0 license, unless otherwise stated. | |
DSSTOX Substance ID |
DTXSID9075377 | |
Record name | Cyclooctane | |
Source | EPA DSSTox | |
URL | https://comptox.epa.gov/dashboard/DTXSID9075377 | |
Description | DSSTox provides a high quality public chemistry resource for supporting improved predictive toxicology. | |
Molecular Weight |
112.21 g/mol | |
Source | PubChem | |
URL | https://pubchem.ncbi.nlm.nih.gov | |
Description | Data deposited in or computed by PubChem | |
Physical Description |
Colorless liquid; mp = 14.8 deg C; [Alfa Aesar MSDS] | |
Record name | Cyclooctane | |
Source | Haz-Map, Information on Hazardous Chemicals and Occupational Diseases | |
URL | https://haz-map.com/Agents/13476 | |
Description | Haz-Map® is an occupational health database designed for health and safety professionals and for consumers seeking information about the adverse effects of workplace exposures to chemical and biological agents. | |
Explanation | Copyright (c) 2022 Haz-Map(R). All rights reserved. Unless otherwise indicated, all materials from Haz-Map are copyrighted by Haz-Map(R). No part of these materials, either text or image may be used for any purpose other than for personal use. Therefore, reproduction, modification, storage in a retrieval system or retransmission, in any form or by any means, electronic, mechanical or otherwise, for reasons other than personal use, is strictly prohibited without prior written permission. | |
CAS No. |
292-64-8 | |
Record name | Cyclooctane | |
Source | CAS Common Chemistry | |
URL | https://commonchemistry.cas.org/detail?cas_rn=292-64-8 | |
Description | CAS Common Chemistry is an open community resource for accessing chemical information. Nearly 500,000 chemical substances from CAS REGISTRY cover areas of community interest, including common and frequently regulated chemicals, and those relevant to high school and undergraduate chemistry classes. This chemical information, curated by our expert scientists, is provided in alignment with our mission as a division of the American Chemical Society. | |
Explanation | The data from CAS Common Chemistry is provided under a CC-BY-NC 4.0 license, unless otherwise stated. | |
Record name | Cyclooctane | |
Source | ChemIDplus | |
URL | https://pubchem.ncbi.nlm.nih.gov/substance/?source=chemidplus&sourceid=0000292648 | |
Description | ChemIDplus is a free, web search system that provides access to the structure and nomenclature authority files used for the identification of chemical substances cited in National Library of Medicine (NLM) databases, including the TOXNET system. | |
Record name | CYCLOOCTANE | |
Source | DTP/NCI | |
URL | https://dtp.cancer.gov/dtpstandard/servlet/dwindex?searchtype=NSC&outputformat=html&searchlist=72426 | |
Description | The NCI Development Therapeutics Program (DTP) provides services and resources to the academic and private-sector research communities worldwide to facilitate the discovery and development of new cancer therapeutic agents. | |
Explanation | Unless otherwise indicated, all text within NCI products is free of copyright and may be reused without our permission. Credit the National Cancer Institute as the source. | |
Record name | Cyclooctane | |
Source | EPA DSSTox | |
URL | https://comptox.epa.gov/dashboard/DTXSID9075377 | |
Description | DSSTox provides a high quality public chemistry resource for supporting improved predictive toxicology. | |
Record name | Cyclooctane | |
Source | European Chemicals Agency (ECHA) | |
URL | https://echa.europa.eu/substance-information/-/substanceinfo/100.005.484 | |
Description | The European Chemicals Agency (ECHA) is an agency of the European Union which is the driving force among regulatory authorities in implementing the EU's groundbreaking chemicals legislation for the benefit of human health and the environment as well as for innovation and competitiveness. | |
Explanation | Use of the information, documents and data from the ECHA website is subject to the terms and conditions of this Legal Notice, and subject to other binding limitations provided for under applicable law, the information, documents and data made available on the ECHA website may be reproduced, distributed and/or used, totally or in part, for non-commercial purposes provided that ECHA is acknowledged as the source: "Source: European Chemicals Agency, http://echa.europa.eu/". Such acknowledgement must be included in each copy of the material. ECHA permits and encourages organisations and individuals to create links to the ECHA website under the following cumulative conditions: Links can only be made to webpages that provide a link to the Legal Notice page. | |
Record name | CYCLOOCTANE | |
Source | FDA Global Substance Registration System (GSRS) | |
URL | https://gsrs.ncats.nih.gov/ginas/app/beta/substances/KKZ3KBS654 | |
Description | The FDA Global Substance Registration System (GSRS) enables the efficient and accurate exchange of information on what substances are in regulated products. Instead of relying on names, which vary across regulatory domains, countries, and regions, the GSRS knowledge base makes it possible for substances to be defined by standardized, scientific descriptions. | |
Explanation | Unless otherwise noted, the contents of the FDA website (www.fda.gov), both text and graphics, are not copyrighted. They are in the public domain and may be republished, reprinted and otherwise used freely by anyone without the need to obtain permission from FDA. Credit to the U.S. Food and Drug Administration as the source is appreciated but not required. | |
Synthesis routes and methods I
Procedure details
Synthesis routes and methods II
Procedure details
Retrosynthesis Analysis
AI-Powered Synthesis Planning: Our tool employs the Template_relevance Pistachio, Template_relevance Bkms_metabolic, Template_relevance Pistachio_ringbreaker, Template_relevance Reaxys, Template_relevance Reaxys_biocatalysis model, leveraging a vast database of chemical reactions to predict feasible synthetic routes.
One-Step Synthesis Focus: Specifically designed for one-step synthesis, it provides concise and direct routes for your target compounds, streamlining the synthesis process.
Accurate Predictions: Utilizing the extensive PISTACHIO, BKMS_METABOLIC, PISTACHIO_RINGBREAKER, REAXYS, REAXYS_BIOCATALYSIS database, our tool offers high-accuracy predictions, reflecting the latest in chemical research and data.
Strategy Settings
Precursor scoring | Relevance Heuristic |
---|---|
Min. plausibility | 0.01 |
Model | Template_relevance |
Template Set | Pistachio/Bkms_metabolic/Pistachio_ringbreaker/Reaxys/Reaxys_biocatalysis |
Top-N result to add to graph | 6 |
Feasible Synthetic Routes
Q1: What is the molecular formula and weight of cyclooctane?
A1: this compound has the molecular formula C8H16 and a molecular weight of 112.21 g/mol.
Q2: How does the this compound ring conformation influence its derivatives?
A3: The size and flexibility of the this compound ring influence the conformation and packing of its derivatives. For example, in cycloalkanespiro-4-imidazolidine-2,5-dithiones, larger cycloalkane rings like this compound exhibit both boat-chair and boat-boat conformations influencing their crystal packing [].
Q3: How does confinement within mesoporous silica nanoparticles (MSNs) affect this compound metathesis?
A4: Confining the reaction within smaller pores of MSNs enhances selectivity towards dimeric cyclohexadecane during this compound metathesis using the WMe6 catalyst. This effect is attributed to the restricted geometry within the pores, limiting the formation of ring-contracted byproducts that are favored in larger pores [].
Q4: Can this compound be used to assess shape-selective effects in zeolite catalysts?
A5: Yes, the bifunctional conversion of this compound is a suitable reaction to test shape selectivity in zeolites. Studies have shown that zeolites with different pore structures (FAU, MFI, and MEL), although chemically identical, exhibit different activities in this compound conversion, with MEL showing the highest activity due to its specific pore dimensions and geometry [].
Q5: How does the structure of a catalyst impact the selectivity of this compound oxidation?
A6: A "helmet" phthalocyaninato iron(III) complex exhibits high selectivity for alcohol formation over ketone formation during cyclohexane and this compound oxidation using hydrogen peroxide. This selectivity arises from the specific steric environment provided by the bulky phthalocyaninato ligand [].
Q6: How does the presence of this compound influence the melting behavior of poly(dimethyl siloxane) (PDMS) precursors and networks?
A7: this compound exhibits anomalous melting behavior in PDMS. Its melting point depression in PDMS networks is lower than in uncrosslinked precursors and is unaffected by the crosslinking density, contradicting typical observations. This behavior suggests phase separation between this compound and PDMS [].
Q7: What computational methods have been employed to study this compound conformations?
A8: Ab initio calculations, particularly at the Hartree-Fock (HF) and Møller-Plesset second-order perturbation theory (MP2) levels, have been extensively used to explore the potential energy surface of this compound, identifying its various conformers and their relative energies []. Molecular dynamics simulations have also provided valuable insights into the conformational dynamics and distributions of this compound in the gas and liquid phases [].
Q8: How do molecular descriptors contribute to understanding this compound derivatives?
A9: Calculating molecular descriptors like the Kirchhoff index, multiplicative degree-Kirchhoff index, and additive degree-Kirchhoff index, based on resistance distances within this compound derivatives, helps understand their structural properties and predict potential physicochemical properties [].
Q9: Can computational methods predict the properties of this compound chains?
A10: Yes, mathematical models have been developed to predict the expected values of various molecular descriptors for random this compound chains. These descriptors, based on atom degrees and sum-degree partitions, offer insights into the structural characteristics of these chains and their potential applications [, ].
Q10: How do structural modifications of this compound derivatives impact their biological activity?
A11: Introducing spiro-annulated or 1,2-annulated this compound rings into 3-[N,N-bis(sulfonyl)amino]isoxazolines leads to promising antiviral activities against tick-borne encephalitis virus (TBEV) and West Nile virus (WNV). The specific substituents on the sulfonyl group significantly influence the potency against different viruses [].
Q11: Can incorporating this compound into pyridoxine derivatives yield antimicrobial agents?
A12: While pyridoxine-adamantane and pyridoxine-cyclooctane dipharmacophores didn't show activity against M. tuberculosis, some exhibited moderate antiviral activity against influenza A virus, comparable to amantadine. This research highlights the potential of these dipharmacophores as a starting point for designing novel anti-infective agents [].
Q12: How can trans-cyclooctane-1,2-dithiol be synthesized?
A14: Three methods are reported for synthesizing trans-cyclooctane-1,2-dithiol: (i) ring-opening of trans-cyclooctene episulfoxide with ammonium thiocyanate followed by reduction; (ii) reduction of trans-1,2-di(thiocyanato)this compound; and (iii) reduction of trans-1,2-cyclooctyl trithiocarbonate [].
Avertissement et informations sur les produits de recherche in vitro
Veuillez noter que tous les articles et informations sur les produits présentés sur BenchChem sont destinés uniquement à des fins informatives. Les produits disponibles à l'achat sur BenchChem sont spécifiquement conçus pour des études in vitro, qui sont réalisées en dehors des organismes vivants. Les études in vitro, dérivées du terme latin "in verre", impliquent des expériences réalisées dans des environnements de laboratoire contrôlés à l'aide de cellules ou de tissus. Il est important de noter que ces produits ne sont pas classés comme médicaments et n'ont pas reçu l'approbation de la FDA pour la prévention, le traitement ou la guérison de toute condition médicale, affection ou maladie. Nous devons souligner que toute forme d'introduction corporelle de ces produits chez les humains ou les animaux est strictement interdite par la loi. Il est essentiel de respecter ces directives pour assurer la conformité aux normes légales et éthiques en matière de recherche et d'expérimentation.