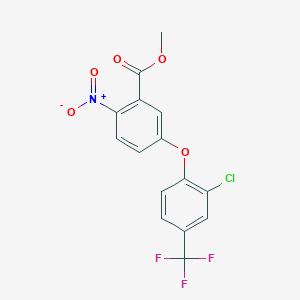
Acifluorfen-méthyle
Vue d'ensemble
Description
Acifluorfen-methyl est un composé chimique principalement utilisé comme herbicide post-émergence. Il est connu pour son efficacité contre les mauvaises herbes à feuilles larges et les graminées. Le composé est généralement utilisé sous sa forme de sel de sodium et est hautement soluble dans l'eau. Acifluorfen-methyl est un dérivé de l'acifluorfen et est reconnu pour ses propriétés de photoblanchiment, qui perturbent le processus photosynthétique dans les plantes .
Applications De Recherche Scientifique
Herbicidal Properties
Acifluorfen-methyl is primarily used as a post-emergent herbicide in various crops, particularly soybeans and peanuts. Its effectiveness lies in its ability to inhibit protoporphyrinogen oxidase (PPO), an enzyme critical for chlorophyll synthesis. This inhibition leads to the accumulation of protoporphyrin IX (PPIX), which subsequently causes photodynamic damage to plant cells when exposed to light.
Table 1: Herbicidal Activity of Acifluorfen-Methyl
Crop | Weed Control Efficacy | Application Timing | Mode of Action |
---|---|---|---|
Soybeans | High | Post-emergent | Inhibition of PPO |
Peanuts | Moderate | Post-emergent | Inhibition of PPO |
Various Broadleaf | Variable | Pre- and Post-emergent | Inhibition of PPO |
Case Study: Phytotoxic Effects on Soybean Cells
In controlled experiments, soybean cells treated with acifluorfen-methyl exhibited increased leakage of potassium ions (86Rb leakage), indicating cellular damage. The degree of damage was correlated with the accumulation of fluorescent pigments characteristic of PPIX, suggesting that the herbicide's toxicity is closely linked to its photodynamic properties .
Environmental Impact and Safety
While acifluorfen-methyl is effective in weed control, its environmental safety profile has been scrutinized. Research indicates that it can act as an endocrine disruptor, affecting various biological pathways in non-target organisms . The potential for bioaccumulation and effects on aquatic ecosystems necessitates careful management and application practices.
Table 2: Environmental Concerns Related to Acifluorfen-Methyl
Concern | Details |
---|---|
Endocrine Disruption | Potential agonistic effects on nuclear receptors |
Aquatic Toxicity | Risk to non-target aquatic organisms |
Bioaccumulation Potential | Possible accumulation in food chains |
Regulatory Status
Acifluorfen-methyl is registered for use in several countries, with specific guidelines regarding application rates and timing to minimize environmental impact. The U.S. Environmental Protection Agency (EPA) has evaluated its safety and efficacy, leading to established tolerances for residues in food crops .
Future Research Directions
Ongoing research is focused on enhancing the specificity and efficacy of acifluorfen-methyl while mitigating its environmental impacts. Studies are exploring:
- Development of formulations that reduce off-target effects.
- Investigation into synergistic effects with other herbicides.
- Long-term ecological studies assessing the impact on soil health and biodiversity.
Mécanisme D'action
Target of Action
Acifluorfen-methyl primarily targets the enzyme protoporphyrinogen oxidase (Protox) . This enzyme plays a crucial role in the biosynthesis of heme and chlorophyll, which are essential components of plant cells .
Mode of Action
Acifluorfen-methyl acts as an inhibitor of Protox . When Protox is inhibited, it leads to the accumulation of the chlorophyll and heme precursor protoporphyrin IX (proto) in plant tissues . This abnormal accumulation of proto is believed to be the primary cause of the herbicidal effects of acifluorfen-methyl .
Biochemical Pathways
The inhibition of Protox by acifluorfen-methyl disrupts the normal biochemical pathways involved in the synthesis of heme and chlorophyll . This disruption leads to the accumulation of proto, which in turn induces lethal photooxidative reactions .
Pharmacokinetics
It is known that acifluorfen-methyl is a protoporphyrinogen oxidase inhibitor . More research is needed to fully understand the ADME (Absorption, Distribution, Metabolism, and Excretion) properties of acifluorfen-methyl and their impact on its bioavailability.
Result of Action
The result of acifluorfen-methyl’s action is the induction of lethal photooxidative reactions in plant cells . These reactions are triggered by the abnormal accumulation of proto, which is caused by the inhibition of Protox . This leads to the death of the plant cells, making acifluorfen-methyl an effective herbicide .
Action Environment
The action of acifluorfen-methyl can be influenced by environmental factors. For instance, it has been shown that the herbicidal effects of acifluorfen-methyl are most pronounced at wavelengths of 350 to 450 nanometers . Additionally, acifluorfen-methyl is highly soluble in water and may be moderately persistent in soils and water depending on conditions . It also has a high potential for leaching to groundwater . These properties suggest that the action, efficacy, and stability of acifluorfen-methyl can be significantly affected by environmental conditions such as light exposure and soil and water composition .
Analyse Biochimique
Biochemical Properties
Acifluorfen-methyl plays a significant role in biochemical reactions by inhibiting the enzyme protoporphyrinogen oxidase (Protox) . This enzyme is crucial for the biosynthesis of heme and chlorophyll. By inhibiting Protox, Acifluorfen-methyl causes the accumulation of protoporphyrin IX, a precursor of chlorophyll and heme . This accumulation leads to the generation of reactive oxygen species (ROS), which cause cellular damage and ultimately lead to the death of the target weeds . The interaction between Acifluorfen-methyl and Protox is a key aspect of its herbicidal activity.
Cellular Effects
Acifluorfen-methyl has profound effects on various types of cells and cellular processes. In nonchlorophyllous soybean cells, it causes the accumulation of tetrapyrroles, which are intermediates in the biosynthesis of chlorophyll and heme . This accumulation leads to photooxidative damage, resulting in cell death . Additionally, Acifluorfen-methyl affects cell signaling pathways, gene expression, and cellular metabolism by inducing the production of ROS . These ROS can damage cellular components, including lipids, proteins, and DNA, leading to impaired cell function and viability.
Molecular Mechanism
The molecular mechanism of Acifluorfen-methyl involves the inhibition of protoporphyrinogen oxidase (Protox), an enzyme involved in the biosynthesis of chlorophyll and heme . By binding to the active site of Protox, Acifluorfen-methyl prevents the conversion of protoporphyrinogen IX to protoporphyrin IX . This inhibition leads to the accumulation of protoporphyrinogen IX, which, in the presence of light, generates ROS . These ROS cause oxidative damage to cellular components, leading to cell death. The inhibition of Protox by Acifluorfen-methyl is a critical step in its herbicidal action.
Temporal Effects in Laboratory Settings
In laboratory settings, the effects of Acifluorfen-methyl change over time. Studies have shown that the herbicide inhibits root elongation in soybean seedlings after 48 hours of exposure, with a more pronounced effect observed after 96 hours . The stability and degradation of Acifluorfen-methyl also play a role in its long-term effects on cellular function. Over time, the accumulation of ROS and the resulting oxidative damage can lead to significant cellular dysfunction and death . These temporal effects highlight the importance of understanding the dynamics of Acifluorfen-methyl’s action in laboratory settings.
Dosage Effects in Animal Models
The effects of Acifluorfen-methyl vary with different dosages in animal models. In mice, high doses of Acifluorfen-methyl (2500 ppm) have been shown to induce liver injury and promote tumor development . The herbicide’s hepatotoxic effects are mediated by the constitutive androstane receptor (CAR), which plays a crucial role in the development of liver tumors in response to certain chemicals . Lower doses of Acifluorfen-methyl may have less severe effects, but the potential for toxicity and adverse effects remains a concern. Understanding the dosage effects of Acifluorfen-methyl is essential for assessing its safety and efficacy in animal models.
Metabolic Pathways
Acifluorfen-methyl is involved in several metabolic pathways, primarily related to its detoxification and bioactivation. In plants, the herbicide is metabolized through a three-phase process involving oxidation, reduction, and hydrolysis . These reactions convert Acifluorfen-methyl into less toxic compounds, which are then conjugated with molecules such as glutathione or amino acids . The final phase involves the formation of secondary conjugates or insoluble bound residues, which are excreted from the plant . The metabolic pathways of Acifluorfen-methyl are crucial for its selectivity and effectiveness as a herbicide.
Transport and Distribution
The transport and distribution of Acifluorfen-methyl within cells and tissues are essential for its herbicidal activity. The herbicide is absorbed by plant leaves and translocated to the target site, where it inhibits Protox . Environmental factors such as temperature and light can influence the uptake and distribution of Acifluorfen-methyl . For example, higher temperatures can alter the composition of the plant cuticle, affecting the herbicide’s penetration and translocation . Understanding the transport and distribution of Acifluorfen-methyl is critical for optimizing its application and efficacy.
Subcellular Localization
Acifluorfen-methyl’s subcellular localization is primarily within the chloroplasts, where it inhibits Protox . The enzyme is located in the inner membrane of the chloroplast, and the herbicide’s binding to Protox prevents the synthesis of chlorophyll . This localization is essential for the herbicide’s mode of action, as it ensures that Acifluorfen-methyl targets the site of chlorophyll biosynthesis. Additionally, the subcellular localization of Acifluorfen-methyl may involve specific targeting signals or post-translational modifications that direct it to the chloroplasts .
Méthodes De Préparation
Voies de synthèse et conditions de réaction
La synthèse de l'acifluorfen-methyl implique plusieurs étapes clés. Les étapes finales comprennent une condensation d'Ullmann entre le 2-chloro-4-trifluorométhylphénol et le 2-nitro-5-fluorobenzonitrile. Cet intermédiaire est ensuite hydrolysé en utilisant de l'acide bromhydrique dans l'acide acétique comme solvant .
Méthodes de production industrielle
Dans les milieux industriels, la production de l'acifluorfen-methyl suit des voies de synthèse similaires, mais à plus grande échelle. Le processus implique un contrôle strict des conditions de réaction pour garantir un rendement et une pureté élevés. Le composé est ensuite formulé en différents produits herbicides pour une utilisation agricole .
Analyse Des Réactions Chimiques
Types de réactions
Acifluorfen-methyl subit plusieurs types de réactions chimiques, notamment :
Oxydation : Le composé peut être oxydé pour former différents métabolites.
Réduction : Il peut être réduit dans des conditions spécifiques pour produire différents produits.
Substitution : Acifluorfen-methyl peut participer à des réactions de substitution, en particulier impliquant ses groupes nitro et chloro.
Réactifs et conditions courants
Oxydation : Les oxydants courants comprennent le peroxyde d'hydrogène et le permanganate de potassium.
Réduction : Des agents réducteurs tels que le borohydrure de sodium et l'hydrure de lithium et d'aluminium sont utilisés.
Substitution : Des réactifs comme l'hydroxyde de sodium et divers solvants organiques facilitent les réactions de substitution.
Principaux produits formés
Les principaux produits formés à partir de ces réactions comprennent l'acifluorfen-amine, l'acifluorfen-acétamide et le des-carboxy-acifluorfen. Ces métabolites sont souvent analysés à l'aide de techniques de chromatographie liquide haute performance (CLHP) et de chromatographie gazeuse (CG) .
Applications de la recherche scientifique
Acifluorfen-methyl a une large gamme d'applications de recherche scientifique :
Chimie : Il est utilisé comme composé modèle pour étudier les mécanismes d'action herbicide et de photoblanchiment.
Biologie : Le composé est utilisé dans la recherche sur la physiologie des plantes et les effets des herbicides sur le métabolisme des plantes.
Médecine : Bien qu'il ne soit pas directement utilisé en médecine, son mécanisme d'action fournit des informations sur le développement de médicaments ciblant des voies similaires.
Mécanisme d'action
Acifluorfen-methyl exerce ses effets herbicides en inhibant l'enzyme protoporphyrinogène oxydase. Cette enzyme est cruciale pour la synthèse de la chlorophylle dans les plantes. L'inhibition entraîne l'accumulation de protoporphyrine IX, un composé phototoxique qui provoque des dommages aux membranes cellulaires lorsqu'il est exposé à la lumière. Cela entraîne le photoblanchiment et la mort éventuelle de la plante .
Comparaison Avec Des Composés Similaires
Composés similaires
Acifluorfen : Le composé parent de l'acifluorfen-methyl, utilisé dans des applications similaires.
Fomesafen : Un autre herbicide à base d'éther diphénylique avec un mécanisme d'action similaire.
Bifenox : Un analogue structural avec un groupe COOCH₃ adjacent au groupe nitro
Unicité
Acifluorfen-methyl est unique en raison de sa haute solubilité dans l'eau et de son efficacité à des concentrations plus faibles par rapport à des composés similaires. Sa capacité à provoquer un photoblanchiment le rend particulièrement efficace contre un large éventail de mauvaises herbes .
Activité Biologique
Acifluorfen-methyl (AFM), a diphenyl ether herbicide, is primarily used in agriculture for weed control. Its biological activity is characterized by its phytotoxic effects on plants, particularly through its interference with chlorophyll biosynthesis and its ability to induce oxidative stress. This article delves into the mechanisms of action, effects on various plant systems, and relevant research findings regarding AFM.
AFM exerts its herbicidal effects primarily through the disruption of chlorophyll biosynthesis. It inhibits the enzyme Mg-chelatase, which is crucial for inserting magnesium into the porphyrin ring of protochlorophyllide. This inhibition leads to an accumulation of protoporphyrin IX, a precursor in the chlorophyll biosynthetic pathway, which subsequently induces photooxidative damage when exposed to light.
Key Findings:
- Inhibition of Protochlorophyllide Synthesis : AFM treatment results in decreased levels of protochlorophyllide and increased levels of protoporphyrin IX in cucumber cotyledons, indicating a disruption in chlorophyll synthesis pathways .
- Photooxidative Stress : The accumulation of protoporphyrin IX acts as a photosensitizer, leading to lipid peroxidation and cellular damage upon light exposure. This process is linked to the generation of reactive oxygen species (ROS) that cause cellular injury .
Phytotoxic Effects
AFM's phytotoxicity has been extensively studied in various plant systems, revealing its effects on non-chlorophyllous cells and its action spectrum.
Action Spectrum Studies:
- Maximum Injury at Specific Wavelengths : Research indicates that AFM causes maximum cellular injury at wavelengths between 350 to 450 nm, with reduced activity observed between 450 and 700 nm. This correlates with the absorption spectrum of protoporphyrin IX .
- Cellular Damage Correlation : The extent of damage in light-exposed cells correlates with the accumulation of fluorescent pigments characteristic of protoporphyrin IX, suggesting that AFM's phytotoxicity is linked to its ability to disrupt normal pigment synthesis .
Case Studies
Several studies have documented the biological activity and effects of AFM on different plant species:
- Cucumber Cotyledons : In experiments with cucumber cotyledons, AFM treatment led to significant increases in protoporphyrin IX levels and subsequent membrane disruption under light conditions. The use of gabaculine (an inhibitor of δ-amino levulinic acid synthesis) demonstrated that blocking this pathway could prevent membrane damage induced by AFM .
- Soybean Cells : A study on non-chlorophyllous soybean cells showed that AFM treatment resulted in increased leakage of potassium ions (measured by Rb leakage), indicating cellular membrane damage. The accumulation of fluorescent pigments was also noted under dark conditions .
Comparative Biological Activity
The following table summarizes key biological activities and effects associated with Acifluorfen-methyl:
Propriétés
IUPAC Name |
methyl 5-[2-chloro-4-(trifluoromethyl)phenoxy]-2-nitrobenzoate | |
---|---|---|
Source | PubChem | |
URL | https://pubchem.ncbi.nlm.nih.gov | |
Description | Data deposited in or computed by PubChem | |
InChI |
InChI=1S/C15H9ClF3NO5/c1-24-14(21)10-7-9(3-4-12(10)20(22)23)25-13-5-2-8(6-11(13)16)15(17,18)19/h2-7H,1H3 | |
Source | PubChem | |
URL | https://pubchem.ncbi.nlm.nih.gov | |
Description | Data deposited in or computed by PubChem | |
InChI Key |
AHGMXAFUHVRQAD-UHFFFAOYSA-N | |
Source | PubChem | |
URL | https://pubchem.ncbi.nlm.nih.gov | |
Description | Data deposited in or computed by PubChem | |
Canonical SMILES |
COC(=O)C1=C(C=CC(=C1)OC2=C(C=C(C=C2)C(F)(F)F)Cl)[N+](=O)[O-] | |
Source | PubChem | |
URL | https://pubchem.ncbi.nlm.nih.gov | |
Description | Data deposited in or computed by PubChem | |
Molecular Formula |
C15H9ClF3NO5 | |
Source | PubChem | |
URL | https://pubchem.ncbi.nlm.nih.gov | |
Description | Data deposited in or computed by PubChem | |
DSSTOX Substance ID |
DTXSID8041583 | |
Record name | Acifluorfen-methyl | |
Source | EPA DSSTox | |
URL | https://comptox.epa.gov/dashboard/DTXSID8041583 | |
Description | DSSTox provides a high quality public chemistry resource for supporting improved predictive toxicology. | |
Molecular Weight |
375.68 g/mol | |
Source | PubChem | |
URL | https://pubchem.ncbi.nlm.nih.gov | |
Description | Data deposited in or computed by PubChem | |
CAS No. |
50594-67-7 | |
Record name | Acifluorfen-methyl [ISO] | |
Source | ChemIDplus | |
URL | https://pubchem.ncbi.nlm.nih.gov/substance/?source=chemidplus&sourceid=0050594677 | |
Description | ChemIDplus is a free, web search system that provides access to the structure and nomenclature authority files used for the identification of chemical substances cited in National Library of Medicine (NLM) databases, including the TOXNET system. | |
Record name | Acifluorfen-methyl | |
Source | EPA DSSTox | |
URL | https://comptox.epa.gov/dashboard/DTXSID8041583 | |
Description | DSSTox provides a high quality public chemistry resource for supporting improved predictive toxicology. | |
Record name | ACIFLUORFEN-METHYL | |
Source | FDA Global Substance Registration System (GSRS) | |
URL | https://gsrs.ncats.nih.gov/ginas/app/beta/substances/9261T4QE1K | |
Description | The FDA Global Substance Registration System (GSRS) enables the efficient and accurate exchange of information on what substances are in regulated products. Instead of relying on names, which vary across regulatory domains, countries, and regions, the GSRS knowledge base makes it possible for substances to be defined by standardized, scientific descriptions. | |
Explanation | Unless otherwise noted, the contents of the FDA website (www.fda.gov), both text and graphics, are not copyrighted. They are in the public domain and may be republished, reprinted and otherwise used freely by anyone without the need to obtain permission from FDA. Credit to the U.S. Food and Drug Administration as the source is appreciated but not required. | |
Synthesis routes and methods I
Procedure details
Synthesis routes and methods II
Procedure details
Synthesis routes and methods III
Procedure details
Retrosynthesis Analysis
AI-Powered Synthesis Planning: Our tool employs the Template_relevance Pistachio, Template_relevance Bkms_metabolic, Template_relevance Pistachio_ringbreaker, Template_relevance Reaxys, Template_relevance Reaxys_biocatalysis model, leveraging a vast database of chemical reactions to predict feasible synthetic routes.
One-Step Synthesis Focus: Specifically designed for one-step synthesis, it provides concise and direct routes for your target compounds, streamlining the synthesis process.
Accurate Predictions: Utilizing the extensive PISTACHIO, BKMS_METABOLIC, PISTACHIO_RINGBREAKER, REAXYS, REAXYS_BIOCATALYSIS database, our tool offers high-accuracy predictions, reflecting the latest in chemical research and data.
Strategy Settings
Precursor scoring | Relevance Heuristic |
---|---|
Min. plausibility | 0.01 |
Model | Template_relevance |
Template Set | Pistachio/Bkms_metabolic/Pistachio_ringbreaker/Reaxys/Reaxys_biocatalysis |
Top-N result to add to graph | 6 |
Feasible Synthetic Routes
Q1: What is the primary molecular target of acifluorfen-methyl in plants?
A1: Acifluorfen-methyl targets the enzyme protoporphyrinogen oxidase (Protox), a key enzyme in the biosynthesis of chlorophyll and heme. [, , , , , ]
Q2: How does acifluorfen-methyl affect Protox activity?
A2: Acifluorfen-methyl acts as a potent inhibitor of Protox. [, , , , , ] This inhibition leads to the accumulation of the enzyme's substrate, protoporphyrinogen IX.
Q3: What are the downstream consequences of Protox inhibition by acifluorfen-methyl?
A3: Protoporphyrinogen IX, in the presence of light and oxygen, is rapidly oxidized to protoporphyrin IX, a photodynamic molecule. [, , , , , , , , , , ] This accumulation of protoporphyrin IX triggers the formation of reactive oxygen species (ROS) which cause damage to cell membranes, ultimately leading to plant death. [, , , , , , , , , ]
Q4: Is light essential for the herbicidal activity of acifluorfen-methyl?
A4: Yes, light is absolutely required for the herbicidal activity of acifluorfen-methyl. [, , , , , , , , , , , , ] The accumulated protoporphyrin IX requires light activation to generate the ROS responsible for cellular damage.
Q5: Are there specific plant pigments involved in the light-activation of acifluorfen-methyl?
A5: Research suggests that carotenoids, and potentially a xanthophyll, play a crucial role in the light-activation mechanism of acifluorfen-methyl. [, , , ] Studies with various chlorophyllous mutants indicate that these pigments are involved in the herbicide's toxicity.
Q6: Does inhibiting carotenoid biosynthesis affect acifluorfen-methyl activity?
A6: Yes, pretreating seedlings with fluridone, a carotenoid biosynthesis inhibitor, provides protection against acifluorfen-methyl injury. [] This further supports the involvement of carotenoids in the light-activation process.
Q7: How rapid is the cellular damage caused by acifluorfen-methyl after light exposure?
A7: Following light exposure, significant increases in membrane permeability, as measured by ion efflux, can be detected within 10 to 15 minutes of acifluorfen-methyl treatment. [, ] This indicates rapid membrane disruption after the light-dependent activation of the herbicide.
Q8: What specific types of cellular damage are observed after acifluorfen-methyl treatment?
A8: Electron microscopy studies reveal significant structural damage to the chloroplast envelope, tonoplast (vacuolar membrane), and plasma membrane in acifluorfen-methyl treated tissues. [] This damage is consistent with the observed increase in membrane permeability and leakage.
Q9: Is there evidence of lipid peroxidation in acifluorfen-methyl treated tissues?
A9: Yes, significant increases in thiobarbituric acid-reacting materials (TBARS), indicative of lipid peroxidation, are detectable within 1 to 2 hours of light exposure in acifluorfen-methyl treated cotyledons. [] This finding further supports the role of ROS in acifluorfen-methyl induced cellular damage.
Q10: Does oxygen play a role in acifluorfen-methyl toxicity?
A10: Yes, acifluorfen-methyl toxicity is reduced in oxygen-deficient atmospheres. [, , ] This supports the involvement of oxygen in the ROS generation pathway triggered by the herbicide.
Q11: Can antioxidants protect against acifluorfen-methyl induced damage?
A11: Yes, pretreatment with α-tocopherol, a potent antioxidant, can protect against acifluorfen-methyl induced injury. [, ] This further supports the role of ROS in the herbicide's mode of action.
Avertissement et informations sur les produits de recherche in vitro
Veuillez noter que tous les articles et informations sur les produits présentés sur BenchChem sont destinés uniquement à des fins informatives. Les produits disponibles à l'achat sur BenchChem sont spécifiquement conçus pour des études in vitro, qui sont réalisées en dehors des organismes vivants. Les études in vitro, dérivées du terme latin "in verre", impliquent des expériences réalisées dans des environnements de laboratoire contrôlés à l'aide de cellules ou de tissus. Il est important de noter que ces produits ne sont pas classés comme médicaments et n'ont pas reçu l'approbation de la FDA pour la prévention, le traitement ou la guérison de toute condition médicale, affection ou maladie. Nous devons souligner que toute forme d'introduction corporelle de ces produits chez les humains ou les animaux est strictement interdite par la loi. Il est essentiel de respecter ces directives pour assurer la conformité aux normes légales et éthiques en matière de recherche et d'expérimentation.