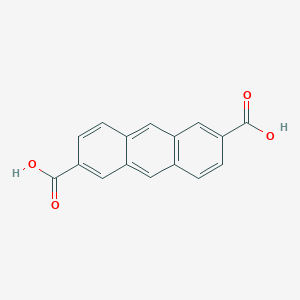
Anthracene-2,6-dicarboxylic acid
Vue d'ensemble
Description
Anthracene-2,6-dicarboxylic acid is a compound with the molecular formula C16H10O4 and a molecular weight of 266.25 . It is a yellow solid and has a larger conjugating π-system that enables the development of fluorescent materials . It also exhibits interesting magnetic and luminescent properties .
Molecular Structure Analysis
The InChI code for Anthracene-2,6-dicarboxylic acid is1S/C16H10O4/c17-15(18)11-3-1-9-5-14-8-12(16(19)20)4-2-10(14)6-13(9)7-11/h1-8H,(H,17,18)(H,19,20)
. This indicates the presence of an anthracene core with carboxylic acid groups at the 2 and 6 positions. Chemical Reactions Analysis
Anthracene-2,6-dicarboxylic acid can undergo a [4+4] photodimerization reaction when irradiated in an aqueous basic solution . This reaction results in the formation of two diastereomeric products .Physical And Chemical Properties Analysis
Anthracene-2,6-dicarboxylic acid is a yellow solid . It has a molecular weight of 266.25 . Unfortunately, other physical and chemical properties such as melting point, boiling point, and density are not available in the retrieved information.Applications De Recherche Scientifique
Formation of Metal Organic Frameworks (MOFs)
Anthracene-2,6-dicarboxylic acid can be used in the formation of highly porous nanotubular and ultramicroporous MOFs . These structures have potential applications in hydrogen storage , methane storage , and gas separation , contributing to greener and more sustainable energy solutions .
Ratiometric Fluorescence Detection
This compound is instrumental in the ratiometric fluorescence detection of anthrax biomarker 2,6-dipicolinic acid (DPA). The detection of DPA is crucial for biosecurity and public health, and Anthracene-2,6-dicarboxylic acid-based MOFs can enhance the sensitivity and specificity of such detection methods .
Enantioselective [4+4] Photodimerization
Anthracene-2,6-dicarboxylic acid undergoes enantioselective [4+4] photodimerization when irradiated in an aqueous basic solution. This reaction leads to the formation of chiral diastereomeric products that have potential applications in the synthesis of new pharmaceuticals and chiral catalysts .
Synthesis of Substituted Anthracene Derivatives
It serves as a precursor for the synthesis of 2,3,6,7-substituted anthracene derivatives . These derivatives are important for developing new organic semiconductors with applications in electronics and photonics .
Safety and Hazards
When handling Anthracene-2,6-dicarboxylic acid, it is advised to avoid dust formation, breathing mist, gas or vapours, and contact with skin and eyes . Use of personal protective equipment and chemical impermeable gloves is recommended . Ensure adequate ventilation and remove all sources of ignition .
Mécanisme D'action
Target of Action
Anthracene-2,6-dicarboxylic acid (ADC) is a compound with interesting magnetic and luminescent properties . It can be used as a bridging carboxylic acid ligand with a steric bulk due to the presence of its anthracene ring
Mode of Action
The mode of action of ADC is primarily through its interaction with light. Specifically, the irradiation of ADC in an aqueous basic solution leads to the formation of two diastereomeric [4+4] photodimerization products . This process is chiral, and the two antipodes are formed in the absence of a chiral control element in a 1:1 ratio . This suggests that the compound’s interaction with its targets and the resulting changes are highly dependent on the presence of light and the specific environmental conditions.
Biochemical Pathways
The biochemical pathways affected by ADC are primarily related to its photodimerization properties. The [4+4] photodimerization of ADC is a key process that leads to the formation of diastereomeric products . This process can be influenced by the presence of a chiral template, which can bind the compound strongly by hydrogen bonding . .
Result of Action
The molecular and cellular effects of ADC’s action are primarily related to its photodimerization properties. The formation of diastereomeric products through [4+4] photodimerization can lead to changes in the compound’s physical and chemical properties . .
Action Environment
The action of ADC is highly dependent on the environmental conditions. For instance, the photodimerization process is influenced by the presence of light and the specific environmental conditions .
Propriétés
IUPAC Name |
anthracene-2,6-dicarboxylic acid | |
---|---|---|
Source | PubChem | |
URL | https://pubchem.ncbi.nlm.nih.gov | |
Description | Data deposited in or computed by PubChem | |
InChI |
InChI=1S/C16H10O4/c17-15(18)11-3-1-9-5-14-8-12(16(19)20)4-2-10(14)6-13(9)7-11/h1-8H,(H,17,18)(H,19,20) | |
Source | PubChem | |
URL | https://pubchem.ncbi.nlm.nih.gov | |
Description | Data deposited in or computed by PubChem | |
InChI Key |
XAAYMWLCUICVSL-UHFFFAOYSA-N | |
Source | PubChem | |
URL | https://pubchem.ncbi.nlm.nih.gov | |
Description | Data deposited in or computed by PubChem | |
Canonical SMILES |
C1=CC(=CC2=CC3=C(C=C21)C=C(C=C3)C(=O)O)C(=O)O | |
Source | PubChem | |
URL | https://pubchem.ncbi.nlm.nih.gov | |
Description | Data deposited in or computed by PubChem | |
Molecular Formula |
C16H10O4 | |
Source | PubChem | |
URL | https://pubchem.ncbi.nlm.nih.gov | |
Description | Data deposited in or computed by PubChem | |
DSSTOX Substance ID |
DTXSID30576884 | |
Record name | Anthracene-2,6-dicarboxylic acid | |
Source | EPA DSSTox | |
URL | https://comptox.epa.gov/dashboard/DTXSID30576884 | |
Description | DSSTox provides a high quality public chemistry resource for supporting improved predictive toxicology. | |
Molecular Weight |
266.25 g/mol | |
Source | PubChem | |
URL | https://pubchem.ncbi.nlm.nih.gov | |
Description | Data deposited in or computed by PubChem | |
Product Name |
Anthracene-2,6-dicarboxylic acid | |
CAS RN |
138308-89-1 | |
Record name | Anthracene-2,6-dicarboxylic acid | |
Source | EPA DSSTox | |
URL | https://comptox.epa.gov/dashboard/DTXSID30576884 | |
Description | DSSTox provides a high quality public chemistry resource for supporting improved predictive toxicology. | |
Retrosynthesis Analysis
AI-Powered Synthesis Planning: Our tool employs the Template_relevance Pistachio, Template_relevance Bkms_metabolic, Template_relevance Pistachio_ringbreaker, Template_relevance Reaxys, Template_relevance Reaxys_biocatalysis model, leveraging a vast database of chemical reactions to predict feasible synthetic routes.
One-Step Synthesis Focus: Specifically designed for one-step synthesis, it provides concise and direct routes for your target compounds, streamlining the synthesis process.
Accurate Predictions: Utilizing the extensive PISTACHIO, BKMS_METABOLIC, PISTACHIO_RINGBREAKER, REAXYS, REAXYS_BIOCATALYSIS database, our tool offers high-accuracy predictions, reflecting the latest in chemical research and data.
Strategy Settings
Precursor scoring | Relevance Heuristic |
---|---|
Min. plausibility | 0.01 |
Model | Template_relevance |
Template Set | Pistachio/Bkms_metabolic/Pistachio_ringbreaker/Reaxys/Reaxys_biocatalysis |
Top-N result to add to graph | 6 |
Feasible Synthetic Routes
Q & A
Q1: What is unique about the photodimerization of Anthracene-2,6-dicarboxylic acid compared to Anthracene-2-carboxylic acid?
A: While both Anthracene-2,6-dicarboxylic acid and Anthracene-2-carboxylic acid can undergo [4+4] photodimerization, the former presents a distinct advantage: the potential for enantioselective control. Anthracene-2,6-dicarboxylic acid possesses two carboxylic acid groups, strategically positioned for interaction with chiral templates. This interaction enables the formation of specific enantiomers (mirror-image isomers) of the photodimer product. In contrast, Anthracene-2-carboxylic acid lacks this dual functionality and its photodimerization typically yields a racemic mixture, which contains equal amounts of both enantiomers [, , ].
Q2: How does the chiral template influence the photodimerization of Anthracene-2,6-dicarboxylic acid?
A: The research highlights the use of a C2-symmetric chiral template designed with two lactam units [, ]. This template selectively binds to Anthracene-2,6-dicarboxylic acid via hydrogen bonding between the template's lactam groups and the acid's carboxylic acid groups. This interaction effectively pre-organizes the Anthracene-2,6-dicarboxylic acid molecules in a specific orientation, favoring the formation of one enantiomer over the other during the photodimerization process. The result is an enantioselective reaction, yielding a product enriched with a specific enantiomer [, ].
Avertissement et informations sur les produits de recherche in vitro
Veuillez noter que tous les articles et informations sur les produits présentés sur BenchChem sont destinés uniquement à des fins informatives. Les produits disponibles à l'achat sur BenchChem sont spécifiquement conçus pour des études in vitro, qui sont réalisées en dehors des organismes vivants. Les études in vitro, dérivées du terme latin "in verre", impliquent des expériences réalisées dans des environnements de laboratoire contrôlés à l'aide de cellules ou de tissus. Il est important de noter que ces produits ne sont pas classés comme médicaments et n'ont pas reçu l'approbation de la FDA pour la prévention, le traitement ou la guérison de toute condition médicale, affection ou maladie. Nous devons souligner que toute forme d'introduction corporelle de ces produits chez les humains ou les animaux est strictement interdite par la loi. Il est essentiel de respecter ces directives pour assurer la conformité aux normes légales et éthiques en matière de recherche et d'expérimentation.