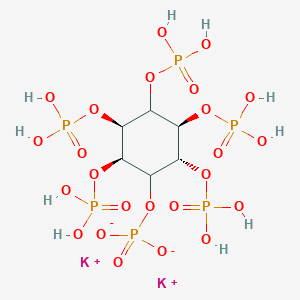
Potassium (1R,2S,3R,4R,5S,6S)-2,3,4,5,6-pentakis(phosphonooxy)cyclohexyl phosphate
Vue d'ensemble
Description
Potassium (1R,2S,3R,4R,5S,6S)-2,3,4,5,6-pentakis(phosphonooxy)cyclohexyl phosphate, commonly known as phytic acid dipotassium salt (CAS: 129832-03-7), is a highly phosphorylated cyclohexanol derivative. Its molecular formula is C₆H₁₆K₂O₂₄P₆, with a molecular weight of 736.22 g/mol . The compound features six phosphate groups attached to a myo-inositol backbone, forming a conformationally stable structure with significant chelating and antioxidant properties. It is primarily used in industrial applications, including metal sequestration, food preservation, and as a precursor in biochemical research .
Mécanisme D'action
Target of Action
Phytic acid dipotassium salt, also known as Potassium (1R,2S,3R,4R,5S,6S)-2,3,4,5,6-pentakis(phosphonooxy)cyclohexyl phosphate, primarily targets the enzyme β-secretase 1 (BACE1) . BACE1 plays a crucial role in the production of beta-amyloid peptide, a key component in the development of Alzheimer’s disease .
Mode of Action
Phytic acid dipotassium salt interacts with BACE1 by inhibiting its activity . The IC50 value, which is the concentration of the compound required to inhibit 50% of the enzyme’s activity, is approximately 0.25 μM . This interaction results in a decrease in the production of beta-amyloid peptide .
Biochemical Pathways
The inhibition of BACE1 by phytic acid dipotassium salt affects the amyloidogenic pathway, which is responsible for the production of beta-amyloid peptide . By inhibiting BACE1, the production of beta-amyloid peptide is reduced, potentially slowing the progression of Alzheimer’s disease .
Result of Action
The primary result of the action of phytic acid dipotassium salt is the reduction in the production of beta-amyloid peptide . This could potentially slow the progression of Alzheimer’s disease .
Action Environment
The action of phytic acid dipotassium salt can be influenced by various environmental factors. For instance, the stability of the compound in cosmetic emulsions was found to decrease with the addition of phytic acid . Additionally, the compound’s action may be affected by the hardness of water in which it is dissolved
Analyse Biochimique
Biochemical Properties
Phytic acid dipotassium salt plays a significant role in biochemical reactions. It is a potent inhibitor of β-secretase 1 (BACE1), an enzyme that plays a key role in the creation of beta-amyloid plaques in the brains of Alzheimer’s patients . The compound’s IC50 value, which measures the effectiveness of a substance in inhibiting a specific biological or biochemical function, is approximately 0.25 μM .
Cellular Effects
Phytic acid dipotassium salt has a profound impact on various types of cells and cellular processes. It is known to function as a storage depot for phosphorus in plant cells . Its high surface negative charge makes it a potent chelator of divalent and trivalent cations in vitro, and it is most likely associated with Ca 2+ or Mg 2+ ions in vivo .
Molecular Mechanism
At the molecular level, Phytic acid dipotassium salt exerts its effects through a variety of mechanisms. It is a potent chelator of divalent and trivalent cations due to its six phosphate groups . This allows it to form strong bonds with these ions, affecting their availability in biological systems .
Temporal Effects in Laboratory Settings
In laboratory settings, the effects of Phytic acid dipotassium salt can change over time. For instance, a colorimetric method for urinary phytate determination, which involves the use of Phytic acid dipotassium salt, has been developed. This method is simple, rapid (3 h for 10 samples), accurate, precise, reliable, and highly sensitive .
Metabolic Pathways
Phytic acid dipotassium salt is involved in several metabolic pathways. In plants, it functions as a storage depot for phosphorus and a precursor for other inositol phosphates and pyrophosphates . The synthesis of phytic acid mainly occurs in the cytosol by two pathways, i.e., lipid dependent and lipid independent manner .
Transport and Distribution
Due to its strong chelating properties, it is likely to interact with various transporters or binding proteins that handle divalent and trivalent metal ions .
Subcellular Localization
The subcellular localization of Phytic acid dipotassium salt is not well studied. Given its role in phosphorus storage in plant cells, it is likely to be found in locations where phosphorus storage occurs, such as vacuoles
Activité Biologique
Potassium (1R,2S,3R,4R,5S,6S)-2,3,4,5,6-pentakis(phosphonooxy)cyclohexyl phosphate is a complex organic compound belonging to the inositol phosphate family. This compound exhibits significant biological activity across various pathways and has garnered attention for its potential therapeutic applications. This article explores its biological activity, mechanisms of action, and relevant research findings.
Structure and Properties
The compound is characterized by its cyclohexane structure with multiple phosphonooxy groups. Its chemical formula is , and it has a molecular weight of approximately 740.015 g/mol. The presence of multiple phosphate groups contributes to its high acidity and reactivity in biological systems.
Biological Activity Overview
- Cell Signaling :
-
Anticancer Properties :
- Recent studies have highlighted the compound's potential as an anticancer agent. It has demonstrated activity against several cancer cell lines including human colon cancer (HT-29) and others. The compound interacts with mitogen-activated protein kinases (MAPKs), influencing cell proliferation and apoptosis pathways .
-
Enzymatic Interactions :
- The compound is involved in enzymatic reactions that regulate phosphate metabolism. It can be biosynthesized from myo-inositol hexakisphosphate and is catalyzed by specific kinases such as inositol hexakisphosphate kinase 1 . This biosynthesis pathway is crucial for maintaining cellular functions under stress conditions.
Case Study 1: Anticancer Activity
A study investigated the effects of this compound on HT-29 colon cancer cells. The findings indicated that the compound inhibited cell growth by inducing apoptosis through the activation of MAPK pathways. The binding energy analysis showed a strong interaction with target proteins involved in cell cycle regulation.
Case Study 2: Calcium Signaling Modulation
In another study focusing on neuronal cells, the compound was found to modulate calcium signaling by acting on IP3 receptors. This modulation resulted in altered neurotransmitter release patterns and enhanced synaptic plasticity. The study utilized electrophysiological techniques to measure changes in intracellular calcium levels upon treatment with the compound.
Research Findings
Study | Findings | Implications |
---|---|---|
Study on HT-29 cells | Inhibition of cell proliferation; induction of apoptosis | Potential therapeutic agent for colorectal cancer |
Neuronal signaling study | Modulation of calcium signaling via IP3 receptors | Implications for neurodegenerative diseases |
Enzymatic activity analysis | Catalysis of phosphate metabolism reactions | Role in cellular stress responses |
Applications De Recherche Scientifique
Plant Growth Promotion
Potassium phytate is recognized as a significant plant growth promoter. It enhances the compatibility of foliar fertilizers with cash crops and improves nutrient uptake. Studies indicate that potassium phytate can enhance photosynthesis and inhibit soil urease activity, leading to better nitrogen retention in soils .
Soil Fertility Improvement
The compound plays a crucial role in improving soil fertility by acting as a slow-release fertilizer. Its interaction with urea slows down decomposition rates and enhances nutrient availability for plants . Moreover, potassium phytate has been shown to improve the bioavailability of phosphorus in soils that are often deficient in this essential nutrient .
Compatibility with Hard Water
Research has highlighted the importance of potassium phytate's resistance to hard water conditions. Its stability in hard water is vital for maintaining fertilizer efficiency and preventing equipment blockage during the spraying process .
Anticancer Properties
Recent studies have identified potassium phytate as a potential anticancer agent due to its ability to interact with key molecular targets involved in cancer progression. Specifically, it has been shown to inhibit β-secretase 1 (BACE1), a target associated with cancer cell proliferation .
Drug-Likeness and Bioactivity
A bioinformatics study assessed the drug-likeness of phytic acid and its analogues. It was found that several derivatives of phytic acid exhibited promising interactions with drug targets such as mitogen-activated protein kinases (MAPKs) and inositol 1,4,5-triphosphate receptors . These interactions suggest potential therapeutic applications in cancer treatment.
Nutritional Characterization
Potassium phytate is also significant in nutritional studies involving seeds and grains. It serves as a major phosphorus reserve in seeds but poses challenges for bioavailability in monogastric animals like poultry due to its indigestibility . This has led to research on methods to enhance phosphorus availability from phytate sources.
Case Study 1: Enhancing Crop Yield
A study conducted on rice crops demonstrated that applying potassium phytate significantly improved crop yield by enhancing nutrient absorption and increasing resistance to pests . The results indicated a marked increase in both yield quantity and quality compared to control groups.
Case Study 2: Phosphorus Utilization in Poultry Feed
Research into poultry feed formulations incorporating potassium phytate showed a reduction in phosphorus excretion by up to 30%, thus mitigating environmental impacts related to eutrophication while also reducing feed costs for farmers .
Q & A
Basic Research Questions
Q. What synthetic methodologies are recommended for synthesizing this compound, and what yields can be expected?
The compound is synthesized via phosphorylation of a cyclohexanol derivative using intermediates like P-triol and o-oxoallyl N,N-diisopropylphosphoramidite. A tetrazole catalyst facilitates coupling, followed by oxidation and dehydration in methanol. Purification via silica gel chromatography yields a 65% isolated product as a colorless solid. Key steps include stereochemical control during phosphorylation and rigorous purification to avoid byproducts .
Q. Which spectroscopic techniques are critical for structural characterization?
- NMR Spectroscopy : P-NMR reveals phosphate group environments, with δ values ranging from -0.21 to -4.38 ppm. H-NMR (CDCl) shows cyclohexyl proton splitting patterns .
- HRMS : High-resolution mass spectrometry confirms molecular ion peaks (e.g., [M+Na] at m/z 1451.1991) .
- IR Spectroscopy : Peaks at 1289.2 cm (P=O stretching) and 1049.1 cm (P-O-C vibrations) validate functional groups .
Q. How does stereochemistry influence binding affinity to biological targets?
The compound’s eight phosphate groups and stereochemical arrangement maximize hydrogen bonding (14 donors, 30 acceptors) and electrostatic interactions. Computational studies (Prime MMGBSA) show strong Coulombic (-38.8 kcal/mol) and van der Waals (-40.1 kcal/mol) contributions, with stereochemistry enabling optimal alignment to binding pockets .
Q. What safety protocols are essential for laboratory handling?
- PPE : Use N95 respirators, gloves, and eye protection .
- First Aid : For skin/eye exposure, rinse with water for 15+ minutes. Avoid inhalation and manage spills with inert absorbents .
- Storage : Maintain under inert atmosphere at 2–8°C to prevent hydrolysis .
Advanced Research Questions
Q. How can discrepancies in computational binding energy predictions be resolved?
Discrepancies arise from force field parameterization (e.g., solvation vs. covalent energy contributions). Validate using multiple methods (e.g., MMGBSA vs. free energy perturbation) and cross-reference experimental data like isothermal titration calorimetry (ITC). Adjust solvation models (e.g., Poisson-Boltzmann vs. Generalized Born) to refine Coulombic (-11092.4 kcal/mol) and solvation energy terms .
Q. What strategies optimize synthetic yield while preserving stereochemical integrity?
- Catalyst Optimization : Replace tetrazole with more efficient activators (e.g., benzimidazolium salts).
- Solvent Screening : Test polar aprotic solvents (e.g., DMF) to enhance reaction kinetics.
- Purification : Use reverse-phase HPLC to isolate stereoisomers, targeting >95% purity .
Q. How can stability under physiological conditions be systematically assessed?
- pH Stability : Incubate in buffers (pH 4–9) and monitor degradation via LC-MS.
- Thermal Stability : Conduct accelerated aging studies (25–40°C) to determine Arrhenius parameters.
- Oxidative Resistance : Expose to reactive oxygen species (HO) and quantify phosphate release .
Q. What analytical thresholds define acceptable purity for research use?
Comparaison Avec Des Composés Similaires
Key Properties :
- Storage : Requires an inert atmosphere and refrigeration (2–8°C) .
- Safety: Classified as non-hazardous for transport but mandates protective equipment (gloves, respirators) during handling .
Comparison with Structurally Similar Compounds
Phytic Acid Analogues
Phytic acid derivatives vary in phosphate group count, stereochemistry, and counterions. Below is a comparative analysis:
Key Observations :
- Phosphate Group Impact : Increasing phosphate groups correlate with higher molecular complexity and binding affinity. The 8-phosphate analogue (ΔG = -53477671 kcal/mol) outperforms the 6-phosphate dipotassium salt in ligand-receptor interactions .
- Solubility and Applications: The dipotassium salt’s potassium counterions enhance water solubility, making it preferable for industrial applications over non-salt forms like myo-inositol hexakisphosphate .
Inositol 1,4,5-Trisphosphate (Ins(1,4,5)P₃)
- Structure: Contains 3 phosphate groups on the inositol ring .
- Role : Key secondary messenger in calcium signaling, unlike the dipotassium salt, which lacks direct signaling functions .
- Binding : Lower phosphate count reduces chelation capacity but increases specificity for intracellular receptors .
Cyclohexyl Phosphate Derivatives
- Example: (2R)-2,3-Bis(octanoyloxy)propyl (1S,2R,3R,4S,5S,6S)-2,3,6-trihydroxy-4,5-bis(phosphonatooxy)cyclohexyl phosphate .
- Differences : Incorporates fatty acid chains, enhancing lipid solubility and membrane permeability. This contrasts with the purely hydrophilic dipotassium salt .
Méthodes De Préparation
Chemical Synthesis from Tert-Butyl Peroxodecanoate Waste
The utilization of industrial byproducts offers a cost-effective route for synthesizing potassium phytate derivatives. A method detailed in recent patents involves repurposing tert-butyl peroxodecanoate waste liquor, which undergoes separation to yield an aqueous phase rich in reactive intermediates . This solution is combined with weathered pulverized coal (80–120 parts) and deionized water (110–200 parts) under vigorous mixing to form a homogeneous slurry .
Heating the mixture to 80–100°C initiates a reaction that progresses over 40–90 minutes, during which the pH rises to 9–11 due to the alkaline nature of pulverized coal . The coal likely acts as a heterogeneous catalyst, facilitating the hydrolysis or oxidative phosphorylation of inositol precursors. Post-reaction, the dark-brown paste is dried at 90–120°C for 2–4 hours, yielding a crude potassium phytate product . While this method minimizes waste, the exact mechanism of inositol phosphorylation remains unclear, necessitating further spectroscopic validation.
Ion Exchange Resin-Mediated Purification
A classical approach to synthesizing water-soluble phytates involves cation exchange resins to replace divalent cations (Ca²⁺, Mg²⁺) in naturally occurring Phytin (calcium magnesium phytate) . The process begins by slurrying Phytin in deionized water, followed by incremental addition of a hydrogen-cycle cation exchange resin until the pH stabilizes between 2–3 . This step displaces Ca²⁺ and Mg²⁺ ions, converting Phytin into phytic acid (InsP₆).
Subsequent neutralization with potassium hydroxide adjusts the pH to 5–8, forming the target potassium salt . Critical purification steps include:
-
Thermal treatment : Heating the solution to ≥65°C for 30 minutes precipitates residual metal phosphates, which are removed via filtration .
-
Decolorization : Activated carbon (1–2% w/v) adsorbs organic impurities, yielding a colorless filtrate .
-
Solvent precipitation : Concentrating the solution under vacuum to a syrup-like consistency and adding anhydrous methanol induces crystallization . The precipitate is washed with cold methanol and dried at 60°C under vacuum, achieving ≥95% purity .
Neutralization and Controlled Crystallization
Adapting techniques from dipotassium phosphate synthesis, this method emphasizes precise neutralization and crystallization control. Phosphoric acid (85% w/w) is diluted with distilled water (1:3 v/v) and titrated with potassium hydroxide until phenolphthalein indicates a pH of 8.2–8.6 . The exothermic reaction requires cooling to maintain temperatures below 80°C during KOH addition .
Post-neutralization, the solution undergoes:
-
Temperature-controlled crystallization : Cooling from 80°C to 0°C at regulated rates (8–10°C/hr initially, slowing to 3–4°C/hr below 55°C) minimizes occluded impurities .
-
Organic solvent partitioning : Introducing ethanol or propyl alcohol (2–3% v/v) and ethyl acetate (4% v/v) at 55–60°C forms a biphasic system, segregating hydrophobic contaminants into the organic layer .
-
Final precipitation : Chilling to 0°C overnight maximizes crystal yield, with vacuum drying at 60°C producing a free-flowing powder .
Comparative Analysis of Preparation Methods
Challenges and Optimization Strategies
Impurity Management
Residual metal ions (Ca²⁺, Fe³⁺) in Phytin-derived routes necessitate stringent cation exchange cycles, as even 10 ppm contaminants can alter crystallization kinetics . Chelating resins (e.g., Dowex MAC-3) show superior selectivity over traditional sulfonated polystyrene resins, reducing potassium loss during ion exchange .
Solvent Selection
Methanol remains the preferred antisolvent for phytate crystallization due to its low polarity, but substituting 20% v/v acetone enhances crystal habit, reducing agglomeration . Ethyl acetate co-solvents improve organic impurity extraction, particularly for lipophilic byproducts from coal-based methods .
pH Control
Maintaining pH >9 during tert-butyl peroxodecanoate reactions prevents premature hydrolysis of phosphate esters . In neutralization routes, automated pH stat systems reduce variability, ensuring consistent product stoichiometry .
Propriétés
IUPAC Name |
dipotassium;[(2R,3S,5R,6R)-2,3,4,5,6-pentaphosphonooxycyclohexyl] phosphate | |
---|---|---|
Source | PubChem | |
URL | https://pubchem.ncbi.nlm.nih.gov | |
Description | Data deposited in or computed by PubChem | |
InChI |
InChI=1S/C6H18O24P6.2K/c7-31(8,9)25-1-2(26-32(10,11)12)4(28-34(16,17)18)6(30-36(22,23)24)5(29-35(19,20)21)3(1)27-33(13,14)15;;/h1-6H,(H2,7,8,9)(H2,10,11,12)(H2,13,14,15)(H2,16,17,18)(H2,19,20,21)(H2,22,23,24);;/q;2*+1/p-2/t1-,2-,3?,4?,5-,6+;;/m0../s1 | |
Source | PubChem | |
URL | https://pubchem.ncbi.nlm.nih.gov | |
Description | Data deposited in or computed by PubChem | |
InChI Key |
LFTTXUFEVNNTHA-OKBOCSEJSA-L | |
Source | PubChem | |
URL | https://pubchem.ncbi.nlm.nih.gov | |
Description | Data deposited in or computed by PubChem | |
Canonical SMILES |
C1(C(C(C(C(C1OP(=O)(O)O)OP(=O)(O)O)OP(=O)([O-])[O-])OP(=O)(O)O)OP(=O)(O)O)OP(=O)(O)O.[K+].[K+] | |
Source | PubChem | |
URL | https://pubchem.ncbi.nlm.nih.gov | |
Description | Data deposited in or computed by PubChem | |
Isomeric SMILES |
[C@H]1([C@@H](C([C@H]([C@@H](C1OP(=O)(O)O)OP(=O)(O)O)OP(=O)(O)O)OP(=O)([O-])[O-])OP(=O)(O)O)OP(=O)(O)O.[K+].[K+] | |
Source | PubChem | |
URL | https://pubchem.ncbi.nlm.nih.gov | |
Description | Data deposited in or computed by PubChem | |
Molecular Formula |
C6H16K2O24P6 | |
Source | PubChem | |
URL | https://pubchem.ncbi.nlm.nih.gov | |
Description | Data deposited in or computed by PubChem | |
DSSTOX Substance ID |
DTXSID801036038 | |
Record name | Phytic acid dipotassium salt | |
Source | EPA DSSTox | |
URL | https://comptox.epa.gov/dashboard/DTXSID801036038 | |
Description | DSSTox provides a high quality public chemistry resource for supporting improved predictive toxicology. | |
Molecular Weight |
736.22 g/mol | |
Source | PubChem | |
URL | https://pubchem.ncbi.nlm.nih.gov | |
Description | Data deposited in or computed by PubChem | |
CAS No. |
129832-03-7 | |
Record name | Phytic acid dipotassium salt | |
Source | EPA DSSTox | |
URL | https://comptox.epa.gov/dashboard/DTXSID801036038 | |
Description | DSSTox provides a high quality public chemistry resource for supporting improved predictive toxicology. | |
Avertissement et informations sur les produits de recherche in vitro
Veuillez noter que tous les articles et informations sur les produits présentés sur BenchChem sont destinés uniquement à des fins informatives. Les produits disponibles à l'achat sur BenchChem sont spécifiquement conçus pour des études in vitro, qui sont réalisées en dehors des organismes vivants. Les études in vitro, dérivées du terme latin "in verre", impliquent des expériences réalisées dans des environnements de laboratoire contrôlés à l'aide de cellules ou de tissus. Il est important de noter que ces produits ne sont pas classés comme médicaments et n'ont pas reçu l'approbation de la FDA pour la prévention, le traitement ou la guérison de toute condition médicale, affection ou maladie. Nous devons souligner que toute forme d'introduction corporelle de ces produits chez les humains ou les animaux est strictement interdite par la loi. Il est essentiel de respecter ces directives pour assurer la conformité aux normes légales et éthiques en matière de recherche et d'expérimentation.