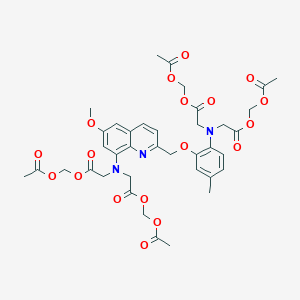
QUIN 2-AM
Vue d'ensemble
Description
QUIN 2-AM (Quin-2 Acetoxymethyl ester) is a cell-permeant fluorescent probe widely used to monitor intracellular free calcium ion (Ca²⁺) concentrations. It is the acetoxymethyl (AM) ester derivative of Quin 2, enabling passive diffusion across cell membranes. Once inside cells, esterases hydrolyze this compound to release the active form, Quin 2, which binds Ca²⁺ with high affinity (logKCaY = 7.1, Kd ≈ 79 nM) . Key properties include:
- Excitation/Emission: 339 nm (ex) and 492 nm (em) in Ca²⁺-bound state .
- Fluorescence Enhancement: 20-fold increase upon Ca²⁺ binding .
- Selectivity: High specificity for Ca²⁺ over Mg²⁺ (logMgY = 2.7) .
- Molecular Formula: C₃₈H₄₃N₃O₁₈, molecular weight 829.8 .
- Storage: Requires -20°C storage in anhydrous DMSO to prevent hydrolysis .
This compound is particularly suited for detecting low Ca²⁺ levels due to its high affinity, but it is sensitive to interference from heavy metals (e.g., Zn²⁺, Fe²⁺), which quench fluorescence unless masked by agents like TPEN .
Méthodes De Préparation
Voies de synthèse et conditions de réaction
Quin-2AM est synthétisé par une série de réactions chimiques impliquant l'estérification de Quin-2 avec des groupes acétoxymethyle. Le processus implique généralement les étapes suivantes :
Estérification : Quin-2 est réagi avec du chlorure d'acétoxymethyle en présence d'une base telle que la triéthylamine pour former Quin-2AM.
Purification : Le produit est purifié en utilisant des techniques telles que la recristallisation ou la chromatographie pour atteindre une pureté élevée
Méthodes de production industrielle
La production industrielle de Quin-2AM suit des voies de synthèse similaires, mais à plus grande échelle. Le processus implique :
Synthèse en vrac : De grandes quantités de Quin-2 sont estérifiées avec du chlorure d'acétoxymethyle.
Purification et contrôle qualité : Le produit subit des mesures rigoureuses de purification et de contrôle qualité pour garantir la cohérence et une pureté élevée
Analyse Des Réactions Chimiques
Types de réactions
Quin-2AM subit principalement des réactions d'hydrolyse et de liaison :
Hydrolyse : Une fois à l'intérieur de la cellule, Quin-2AM est hydrolysé par des estérases intracellulaires pour libérer Quin-2.
Liaison au calcium : Quin-2 lie les ions calcium avec une forte affinité, ce qui entraîne un changement significatif de ses propriétés de fluorescence
Réactifs et conditions courants
Hydrolyse : Les estérases intracellulaires sont les principaux agents d'hydrolyse.
Liaison au calcium : La liaison se produit dans des conditions physiologiques, Quin-2 affichant une grande sélectivité pour le calcium par rapport à d'autres ions tels que le magnésium
Principaux produits formés
Produit d'hydrolyse : Quin-2.
Produit de liaison au calcium : Complexe Quin-2-calcium
Applications de la recherche scientifique
Quin-2AM a un large éventail d'applications dans la recherche scientifique :
Chimie : Utilisé comme indicateur fluorescent pour les ions calcium dans diverses analyses chimiques.
Biologie : Essentiel pour l'étude des voies de signalisation du calcium dans les cellules.
Médecine : Utilisé dans la recherche sur les maladies et les affections liées au calcium.
Industrie : Appliqué dans le développement de matériaux et de capteurs sensibles au calcium .
Mécanisme d'action
Quin-2AM exerce ses effets par le mécanisme suivant :
Perméation cellulaire : Quin-2AM diffuse dans les cellules en raison de sa nature perméable aux cellules.
Hydrolyse : Les estérases intracellulaires hydrolysent Quin-2AM pour libérer Quin-2.
Liaison au calcium : Quin-2 lie les ions calcium, ce qui entraîne une augmentation significative de la fluorescence. .
Applications De Recherche Scientifique
Calcium Imaging
Fluorescence Lifetime Imaging (FLIM)
Quin-2-AM has been utilized in advanced imaging techniques such as fluorescence lifetime imaging (FLIM). This method allows researchers to visualize calcium concentrations within cells without the need for shifts in excitation or emission spectra. The lifetime of Quin-2 fluorescence changes depending on the binding of calcium ions, enabling precise measurements of intracellular calcium levels .
Case Study: Neuronal Calcium Imaging
In a study focused on neuronal cells, Quin-2-AM was employed to assess intracellular calcium dynamics. The FLIM technique demonstrated that Quin-2-AM could effectively distinguish between different calcium concentrations based on fluorescence lifetimes, providing insights into neuronal signaling mechanisms .
Comparison with Other Calcium Indicators
Fura-2 vs. Quin-2
While Quin-2-AM is a valuable tool, it is often compared with other indicators like Fura-2. A study highlighted that Fura-2, which requires dual excitation wavelengths, tends to yield more accurate measurements of synaptosomal calcium levels than Quin-2-AM, which may underestimate calcium concentrations due to dye chelation with trace elements. However, Quin-2-AM's simplicity and lower photobleaching rates make it advantageous for certain applications .
Indicator | Advantages | Disadvantages |
---|---|---|
Quin-2-AM | Simple loading, minimal interference from Mg²⁺ | Potential underestimation of [Ca²⁺] due to chelation |
Fura-2 | More accurate measurements, ratiometric analysis | Requires dual excitation wavelengths |
Applications in Platelet Studies
Quin-2-AM has been utilized to study cytosolic calcium levels in platelets. In one experiment, washed platelets were treated with Quin-2-AM and analyzed using fluorescence microscopy. The results indicated that Quin-2-AM could effectively visualize calcium mobilization in response to ADP stimulation, highlighting its utility in hematological research .
Investigating Cellular Responses
Calcium Dynamics in Mitochondria
Research has shown that Quin-2-AM can also be applied to investigate mitochondrial calcium uptake. In isolated mitochondria studies, Quin-2-AM was used to measure free calcium concentrations, revealing important insights into mitochondrial function and its role in cellular metabolism .
Limitations and Considerations
Despite its advantages, researchers must consider the limitations of Quin-2-AM. The probe's sensitivity to trace elements can lead to inaccuracies in low-calcium environments. Additionally, while it provides valuable data on calcium dynamics, its use may be limited by the requirement for specific experimental conditions, such as optimal dye concentration and loading times .
Mécanisme D'action
Quin-2AM exerts its effects through the following mechanism:
Comparaison Avec Des Composés Similaires
Comparison with Similar Calcium Ion Probes
Key Properties of Common Ca²⁺ Probes
Probe | Excitation (nm) | Emission (nm) | Kd (nM) | Dynamic Range (Fluo. Increase) | Key Applications | Interference Considerations |
---|---|---|---|---|---|---|
QUIN 2-AM | 339 | 492 | ~79 | 20× | Low Ca²⁺ detection, static assays | Heavy metals (requires TPEN masking) |
Fura 2-AM | 340/380 (ratio) | 500 | 224 | Ratiometric | Ratiometric imaging, dynamic Ca²⁺ | UV photobleaching, filter switching |
Fluo 3-AM | 506 | 526 | ~400 | 100× | High Ca²⁺ levels, live-cell imaging | pH sensitivity, Mg²⁺ interference |
Indo 1-AM | 349 | 405/485 | 250 | Ratiometric | Ratiometric emission shifts | Overlap in emission wavelengths |
Rhod 2-AM | 552 | 581 | ~570 | Moderate | Mitochondrial Ca²⁺, high-affinity | Background autofluorescence |
Detailed Comparative Analysis
This compound vs. Fura 2-AM
- Affinity and Sensitivity : this compound has a higher Ca²⁺ affinity (Kd ~79 nM vs. 224 nM for Fura 2-AM), making it ideal for low Ca²⁺ environments. However, Fura 2-AM’s ratiometric dual-excitation (340/380 nm) allows precise quantification independent of probe concentration .
- Practical Considerations : Fura 2-AM requires UV excitation and filter switching, which may limit temporal resolution. This compound’s single-wavelength detection simplifies setup but lacks intrinsic calibration .
This compound vs. Fluo 3-AM
- Dynamic Range : Fluo 3-AM exhibits a 100× fluorescence increase upon Ca²⁺ binding, outperforming this compound’s 20× enhancement. This makes Fluo 3-AM preferable for detecting rapid Ca²⁺ transients in live cells .
- Affinity Trade-offs : Fluo 3-AM’s lower affinity (Kd ~400 nM) suits higher Ca²⁺ concentrations (e.g., during cellular activation), whereas this compound may buffer Ca²⁺ due to its high affinity, altering physiological signals .
This compound vs. Indo 1-AM
- Ratiometric Capability : Indo 1-AM provides ratiometric emission shifts (405/485 nm), similar to Fura 2-AM, but overlaps in emission wavelengths complicate data interpretation. This compound’s single emission simplifies detection but lacks self-calibration .
- Interference : Indo 1-AM is less affected by heavy metals, whereas this compound requires masking agents like TPEN to avoid fluorescence quenching .
This compound vs. Rhod 2-AM
- Localization : Rhod 2-AM accumulates in mitochondria due to its positive charge, enabling organelle-specific Ca²⁺ measurements. This compound distributes uniformly in the cytosol .
- Excitation Wavelengths : Rhod 2-AM’s longer excitation (552 nm) reduces autofluorescence in tissue samples, whereas this compound’s UV excitation may damage cells during prolonged imaging .
Research Findings and Practical Considerations
- Heavy Metal Interference: this compound’s fluorescence is quenched by Zn²⁺ and Fe²⁺, necessitating the use of TPEN (a non-fluorescent chelator) to mask these ions .
- Handling Challenges : this compound’s hygroscopic nature and rapid hydrolysis require strict storage conditions (-20°C in anhydrous DMSO) and immediate use after reconstitution .
Activité Biologique
Quin 2-AM, a membrane-permeable derivative of the calcium chelator Quin 2, has garnered attention in biological research due to its ability to modulate intracellular calcium levels. This article explores the biological activity of this compound, focusing on its mechanisms of action, applications in cellular studies, and implications for understanding calcium signaling pathways.
Overview of this compound
This compound is a fluorescent calcium indicator that is utilized primarily for monitoring intracellular calcium concentrations. It functions by binding to calcium ions (Ca²⁺), allowing researchers to visualize and quantify changes in calcium levels within cells. The compound is particularly valuable in studies involving cellular signaling, muscle contraction, and neuronal activity.
This compound operates through several mechanisms that influence intracellular calcium dynamics:
- Calcium Binding : this compound binds to free Ca²⁺ ions, altering its fluorescence properties. This change can be detected using fluorescence microscopy, providing real-time monitoring of calcium fluctuations within cells .
- Calcium Chelation : As a chelator, this compound can sequester Ca²⁺ ions, thereby reducing their availability for signaling pathways. This property has been shown to prevent oxidative stress-induced DNA damage in cellular models .
- Impact on Cellular Responses : By modulating intracellular calcium levels, this compound affects various physiological responses, including muscle contraction and neurotransmitter release. For instance, studies have demonstrated that this compound can significantly reduce cellular toxicity induced by oxidative agents like hydrogen peroxide .
Research Findings
Numerous studies have investigated the biological activity of this compound, highlighting its significance in various contexts:
Table 1: Summary of Key Studies on this compound
Case Study: Protective Effects Against Oxidative Stress
In a pivotal study involving Chinese hamster ovary (CHO) cells, researchers found that treatment with this compound significantly reduced DNA damage caused by oxidative stress from hydrogen peroxide exposure. The protective effect was noted at concentrations as low as 0.1 µM, with maximal effects observed at 1 µM. Importantly, the study indicated that extracellular calcium chelators did not influence the DNA breakage caused by hydrogen peroxide, underscoring the specific role of intracellular calcium modulation by this compound .
Case Study: Calcium Imaging in Neuronal Activity
Another notable application of this compound was in neuronal studies where it was employed to visualize calcium transients during synaptic activity. The ability to monitor real-time changes in intracellular calcium provided insights into the mechanisms underlying neurotransmitter release and synaptic plasticity. This research has implications for understanding neurological disorders where calcium signaling is disrupted .
Q & A
Basic Research Questions
Q. What experimental methodologies are recommended for verifying the intracellular hydrolysis of QUIN 2-AM in live-cell calcium imaging?
To confirm hydrolysis, researchers should combine fluorescence microscopy with esterase inhibition controls. For example, pre-treating cells with esterase inhibitors (e.g., phenylmethylsulfonyl fluoride) and comparing fluorescence intensity before/after treatment can validate hydrolysis efficiency. Parallel calibration using calcium ionophores (e.g., ionomycin) ensures proper intracellular retention of the hydrolyzed QUIN 2 . Additionally, mass spectrometry can detect residual ester forms post-incubation .
Q. How can researchers optimize this compound loading concentrations to avoid cytotoxicity while maintaining signal fidelity?
Titration experiments are critical. Start with a concentration range of 1–10 µM and assess viability via MTT assays. Fluorescence intensity should be measured using microplate readers or confocal microscopy to identify the lowest effective concentration. Note that prolonged incubation (>30 minutes) may induce cytotoxicity; thus, time-course studies are recommended .
Q. What controls are essential for validating this compound specificity in calcium flux experiments?
Include:
- Negative controls: Calcium-free buffers with chelators (EGTA) to confirm signal reduction.
- Positive controls: Calcium ionophores (e.g., A23187) to saturate the indicator.
- Interference controls: Test for cross-reactivity with magnesium or other divalent cations using buffers with elevated Mg²⁺ .
Advanced Research Questions
Q. How should researchers address discrepancies in this compound’s reported dissociation constant (Kd) across different cell types?
Variability in Kd (~60–115 nM) often stems from differences in intracellular pH, temperature, or ionic strength. To resolve this:
- Calibrate Kd in situ using calcium buffers (e.g., Calcium Calibration Buffer Kit) under physiologically relevant conditions.
- Perform ratiometric measurements (if equipment permits) to normalize signal artifacts.
- Compare results with alternative indicators (e.g., Fura-2) for cross-validation .
Q. What statistical approaches are suitable for analyzing time-lapse calcium oscillations captured via this compound?
Use Fourier transform analysis or wavelet decomposition to quantify oscillation frequency and amplitude. For non-periodic signals, employ peak detection algorithms (e.g., threshold-based or derivative methods) paired with bootstrapping to assess confidence intervals. Normalize data to baseline fluorescence (F/F₀) to account for photobleaching .
Q. How can researchers reconcile conflicting data on this compound’s compartmentalization in organelles versus cytosol?
Subcellular localization can be confirmed via:
- Co-staining with organelle-specific dyes (e.g., MitoTracker for mitochondria).
- Fractionation assays (e.g., differential centrifugation) followed by fluorescence quantification.
- Use of targeted esterase inhibitors (e.g., organelle-specific esterase blockers) .
Q. What experimental designs mitigate phototoxicity during prolonged this compound imaging?
- Use low-intensity illumination and optimize exposure times via preliminary photobleaching curves.
- Employ oxygen scavenger systems (e.g., glucose oxidase/catalase) to reduce reactive oxygen species.
- Validate cell viability post-imaging using propidium iodide or annexin V assays .
Q. Methodological Best Practices
Q. How should researchers design a calibration curve for this compound in heterogeneous cell populations?
- Generate cell-specific calibration curves using calcium ionophores and buffers.
- Account for cell-to-cell variability by analyzing fluorescence distributions (e.g., kernel density plots) rather than population averages.
- Use microfluidic devices to create controlled calcium gradients for high-resolution calibration .
Q. What protocols ensure reproducibility in this compound-based calcium flux assays across laboratories?
- Standardize buffer composition (pH 7.2–7.4, 1–2 mM Mg²⁺).
- Document temperature (e.g., 37°C vs. room temperature) and loading duration.
- Share raw fluorescence data and metadata via repositories like Zenodo to facilitate cross-lab comparisons .
Q. Addressing Data Contradictions
Q. How can researchers resolve conflicts between this compound data and electrophysiological recordings of calcium currents?
Discrepancies may arise from this compound’s slow binding kinetics relative to rapid calcium transients. Solutions include:
- Pairing with high-temporal-resolution methods (e.g., patch-clamp with fluorescence同步 recording).
- Applying kinetic modeling to deconvolve indicator lag from true calcium dynamics .
Q. What steps validate this compound’s utility in pathological models with altered membrane integrity?
In cells with compromised membranes (e.g., necrosis), use:
Propriétés
IUPAC Name |
acetyloxymethyl 2-[N-[2-(acetyloxymethoxy)-2-oxoethyl]-2-[[8-[bis[2-(acetyloxymethoxy)-2-oxoethyl]amino]-6-methoxyquinolin-2-yl]methoxy]-4-methylanilino]acetate | |
---|---|---|
Source | PubChem | |
URL | https://pubchem.ncbi.nlm.nih.gov | |
Description | Data deposited in or computed by PubChem | |
InChI |
InChI=1S/C38H43N3O18/c1-23-7-10-31(40(14-34(46)56-19-52-24(2)42)15-35(47)57-20-53-25(3)43)33(11-23)51-18-29-9-8-28-12-30(50-6)13-32(38(28)39-29)41(16-36(48)58-21-54-26(4)44)17-37(49)59-22-55-27(5)45/h7-13H,14-22H2,1-6H3 | |
Source | PubChem | |
URL | https://pubchem.ncbi.nlm.nih.gov | |
Description | Data deposited in or computed by PubChem | |
InChI Key |
ANRZUBSJAOAXHS-UHFFFAOYSA-N | |
Source | PubChem | |
URL | https://pubchem.ncbi.nlm.nih.gov | |
Description | Data deposited in or computed by PubChem | |
Canonical SMILES |
CC1=CC(=C(C=C1)N(CC(=O)OCOC(=O)C)CC(=O)OCOC(=O)C)OCC2=NC3=C(C=C(C=C3C=C2)OC)N(CC(=O)OCOC(=O)C)CC(=O)OCOC(=O)C | |
Source | PubChem | |
URL | https://pubchem.ncbi.nlm.nih.gov | |
Description | Data deposited in or computed by PubChem | |
Molecular Formula |
C38H43N3O18 | |
Source | PubChem | |
URL | https://pubchem.ncbi.nlm.nih.gov | |
Description | Data deposited in or computed by PubChem | |
DSSTOX Substance ID |
DTXSID10232173 | |
Record name | Quin2-acetoxymethyl ester | |
Source | EPA DSSTox | |
URL | https://comptox.epa.gov/dashboard/DTXSID10232173 | |
Description | DSSTox provides a high quality public chemistry resource for supporting improved predictive toxicology. | |
Molecular Weight |
829.8 g/mol | |
Source | PubChem | |
URL | https://pubchem.ncbi.nlm.nih.gov | |
Description | Data deposited in or computed by PubChem | |
CAS No. |
83104-85-2 | |
Record name | Quin2-acetoxymethyl ester | |
Source | ChemIDplus | |
URL | https://pubchem.ncbi.nlm.nih.gov/substance/?source=chemidplus&sourceid=0083104852 | |
Description | ChemIDplus is a free, web search system that provides access to the structure and nomenclature authority files used for the identification of chemical substances cited in National Library of Medicine (NLM) databases, including the TOXNET system. | |
Record name | Quin2-acetoxymethyl ester | |
Source | EPA DSSTox | |
URL | https://comptox.epa.gov/dashboard/DTXSID10232173 | |
Description | DSSTox provides a high quality public chemistry resource for supporting improved predictive toxicology. | |
Record name | Quin� 2-AM | |
Source | European Chemicals Agency (ECHA) | |
URL | https://echa.europa.eu/information-on-chemicals | |
Description | The European Chemicals Agency (ECHA) is an agency of the European Union which is the driving force among regulatory authorities in implementing the EU's groundbreaking chemicals legislation for the benefit of human health and the environment as well as for innovation and competitiveness. | |
Explanation | Use of the information, documents and data from the ECHA website is subject to the terms and conditions of this Legal Notice, and subject to other binding limitations provided for under applicable law, the information, documents and data made available on the ECHA website may be reproduced, distributed and/or used, totally or in part, for non-commercial purposes provided that ECHA is acknowledged as the source: "Source: European Chemicals Agency, http://echa.europa.eu/". Such acknowledgement must be included in each copy of the material. ECHA permits and encourages organisations and individuals to create links to the ECHA website under the following cumulative conditions: Links can only be made to webpages that provide a link to the Legal Notice page. | |
Record name | QUIN2 ACETOXYMETHYL ESTER | |
Source | FDA Global Substance Registration System (GSRS) | |
URL | https://gsrs.ncats.nih.gov/ginas/app/beta/substances/8EF0C5H59D | |
Description | The FDA Global Substance Registration System (GSRS) enables the efficient and accurate exchange of information on what substances are in regulated products. Instead of relying on names, which vary across regulatory domains, countries, and regions, the GSRS knowledge base makes it possible for substances to be defined by standardized, scientific descriptions. | |
Explanation | Unless otherwise noted, the contents of the FDA website (www.fda.gov), both text and graphics, are not copyrighted. They are in the public domain and may be republished, reprinted and otherwise used freely by anyone without the need to obtain permission from FDA. Credit to the U.S. Food and Drug Administration as the source is appreciated but not required. | |
Retrosynthesis Analysis
AI-Powered Synthesis Planning: Our tool employs the Template_relevance Pistachio, Template_relevance Bkms_metabolic, Template_relevance Pistachio_ringbreaker, Template_relevance Reaxys, Template_relevance Reaxys_biocatalysis model, leveraging a vast database of chemical reactions to predict feasible synthetic routes.
One-Step Synthesis Focus: Specifically designed for one-step synthesis, it provides concise and direct routes for your target compounds, streamlining the synthesis process.
Accurate Predictions: Utilizing the extensive PISTACHIO, BKMS_METABOLIC, PISTACHIO_RINGBREAKER, REAXYS, REAXYS_BIOCATALYSIS database, our tool offers high-accuracy predictions, reflecting the latest in chemical research and data.
Strategy Settings
Precursor scoring | Relevance Heuristic |
---|---|
Min. plausibility | 0.01 |
Model | Template_relevance |
Template Set | Pistachio/Bkms_metabolic/Pistachio_ringbreaker/Reaxys/Reaxys_biocatalysis |
Top-N result to add to graph | 6 |
Feasible Synthetic Routes
Avertissement et informations sur les produits de recherche in vitro
Veuillez noter que tous les articles et informations sur les produits présentés sur BenchChem sont destinés uniquement à des fins informatives. Les produits disponibles à l'achat sur BenchChem sont spécifiquement conçus pour des études in vitro, qui sont réalisées en dehors des organismes vivants. Les études in vitro, dérivées du terme latin "in verre", impliquent des expériences réalisées dans des environnements de laboratoire contrôlés à l'aide de cellules ou de tissus. Il est important de noter que ces produits ne sont pas classés comme médicaments et n'ont pas reçu l'approbation de la FDA pour la prévention, le traitement ou la guérison de toute condition médicale, affection ou maladie. Nous devons souligner que toute forme d'introduction corporelle de ces produits chez les humains ou les animaux est strictement interdite par la loi. Il est essentiel de respecter ces directives pour assurer la conformité aux normes légales et éthiques en matière de recherche et d'expérimentation.