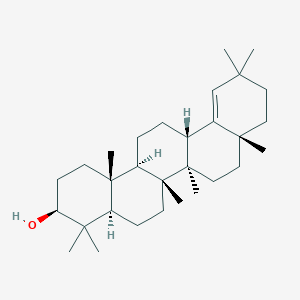
Germanicol
Vue d'ensemble
Description
Germanicol is a pentacyclic triterpenoid that belongs to the oleanane class of compounds. It is characterized by a hydroxy group at the 3β-position and a double bond between positions 18 and 19 . This compound is naturally found in various plant species and has been studied for its potential biological activities, including anticancer, anti-inflammatory, and antioxidant properties .
Applications De Recherche Scientifique
Mécanisme D'action
Target of Action
Germanicol, a natural pentacyclic triterpenoid , has been found to exhibit selective antineoplastic activity against human colon cancer cell lines HCT-116 and HT29 . The primary targets of this compound are these cancer cells, particularly their processes of growth and migration .
Mode of Action
This compound interacts with its targets, the HCT-116 and HT29 colon cancer cells, by inducing apoptosis, a process of programmed cell death . This is achieved through chromatin condensation and DNA damage . The compound’s interaction with these cells results in potent and dose-dependent cytotoxicity .
Biochemical Pathways
The biochemical pathways affected by this compound involve the induction of apoptosis in the target cells . This process is characterized by chromatin condensation and DNA damage
Result of Action
The result of this compound’s action is the selective, potent, and dose-dependent inhibition of growth in HCT-116 and HT29 human colon cancer cells . It also induces cell death in these cells in a dose-dependent manner . Furthermore, this compound inhibits the migration of HCT-116 colon cancer cells .
Analyse Biochimique
Biochemical Properties
Germanicol interacts with various biomolecules in human colon cancer cells, leading to chromatin condensation and DNA damage . This interaction triggers apoptosis, a form of programmed cell death .
Cellular Effects
This compound has a potent and dose-dependent cytotoxic effect on HCT-116 and HT29 human colon cancer cells . It induces apoptosis and inhibits cell migration , impacting cell signaling pathways, gene expression, and cellular metabolism.
Molecular Mechanism
At the molecular level, this compound exerts its effects through binding interactions with biomolecules, leading to chromatin condensation and DNA damage . This results in the activation of apoptosis and inhibition of cell migration .
Temporal Effects in Laboratory Settings
Over time, this compound continues to show selective, potent, and dose-dependent cytotoxicity in HCT-116 and HT29 human colon cancer cells
Méthodes De Préparation
Synthetic Routes and Reaction Conditions: The synthesis of germanicol can be achieved through a biomimetic approach, which involves constructing the ring systems in a manner similar to natural biosynthesis. One such method starts with farnesyl acetate, which undergoes Corey-Zhang oxidation to form a diene intermediate. This intermediate is then subjected to a series of reactions, including the addition of 2-propenyllithium, a Brook rearrangement, and trapping with an aryl bromide . The key step involves a cationic cyclization promoted by a Lewis acid, leading to the formation of the pseudo A, B, and C rings .
Industrial Production Methods: Industrial production of this compound typically involves extraction from plant sources, such as the leaves of various Ficus species. High-performance thin-layer chromatography (HPTLC) is used to estimate the concentration of this compound in these plant extracts . The extraction process involves the use of solvents like hexane and chloroform, followed by derivatization with p-anisaldehyde reagent to obtain well-resolved and compact spots of this compound .
Analyse Des Réactions Chimiques
Types of Reactions: Germanicol undergoes various chemical reactions, including oxidation, reduction, and substitution. For instance, it can be oxidized to form this compound acetate or other derivatives .
Common Reagents and Conditions:
Oxidation: Common oxidizing agents like potassium permanganate or chromium trioxide can be used to oxidize this compound.
Reduction: Reducing agents such as lithium aluminum hydride can be employed to reduce this compound derivatives.
Substitution: Substitution reactions can be carried out using reagents like halogens or alkylating agents.
Major Products Formed: The major products formed from these reactions include this compound acetate, this compound caffeoyl ester, and this compound coumaroyl esters .
Comparaison Avec Des Composés Similaires
Betulinic Acid: Another pentacyclic triterpenoid with similar anticancer properties.
Oleanolic Acid: Shares structural similarities with germanicol and exhibits anti-inflammatory and hepatoprotective effects.
Ursolic Acid: Known for its antioxidant and anticancer activities.
Uniqueness of this compound: this compound is unique due to its specific structural features, such as the hydroxy group at the 3β-position and the double bond between positions 18 and 19 . These structural attributes contribute to its distinct biological activities and make it a valuable compound for further research and development.
Propriétés
IUPAC Name |
(3S,4aR,6aS,6aR,6bR,8aR,14aR,14bR)-4,4,6a,6b,8a,11,11,14b-octamethyl-1,2,3,4a,5,6,6a,7,8,9,10,13,14,14a-tetradecahydropicen-3-ol | |
---|---|---|
Source | PubChem | |
URL | https://pubchem.ncbi.nlm.nih.gov | |
Description | Data deposited in or computed by PubChem | |
InChI |
InChI=1S/C30H50O/c1-25(2)15-16-27(5)17-18-29(7)20(21(27)19-25)9-10-23-28(6)13-12-24(31)26(3,4)22(28)11-14-30(23,29)8/h19-20,22-24,31H,9-18H2,1-8H3/t20-,22+,23-,24+,27-,28+,29-,30-/m1/s1 | |
Source | PubChem | |
URL | https://pubchem.ncbi.nlm.nih.gov | |
Description | Data deposited in or computed by PubChem | |
InChI Key |
QMUXVPRGNJLGRT-PNTWTTAKSA-N | |
Source | PubChem | |
URL | https://pubchem.ncbi.nlm.nih.gov | |
Description | Data deposited in or computed by PubChem | |
Canonical SMILES |
CC1(CCC2(CCC3(C(C2=C1)CCC4C3(CCC5C4(CCC(C5(C)C)O)C)C)C)C)C | |
Source | PubChem | |
URL | https://pubchem.ncbi.nlm.nih.gov | |
Description | Data deposited in or computed by PubChem | |
Isomeric SMILES |
C[C@@]12CC[C@@]3([C@@H](C1=CC(CC2)(C)C)CC[C@H]4[C@]3(CC[C@@H]5[C@@]4(CC[C@@H](C5(C)C)O)C)C)C | |
Source | PubChem | |
URL | https://pubchem.ncbi.nlm.nih.gov | |
Description | Data deposited in or computed by PubChem | |
Molecular Formula |
C30H50O | |
Source | PubChem | |
URL | https://pubchem.ncbi.nlm.nih.gov | |
Description | Data deposited in or computed by PubChem | |
DSSTOX Substance ID |
DTXSID00963585 | |
Record name | Olean-18-en-3-ol | |
Source | EPA DSSTox | |
URL | https://comptox.epa.gov/dashboard/DTXSID00963585 | |
Description | DSSTox provides a high quality public chemistry resource for supporting improved predictive toxicology. | |
Molecular Weight |
426.7 g/mol | |
Source | PubChem | |
URL | https://pubchem.ncbi.nlm.nih.gov | |
Description | Data deposited in or computed by PubChem | |
CAS No. |
465-02-1 | |
Record name | Germanicol | |
Source | CAS Common Chemistry | |
URL | https://commonchemistry.cas.org/detail?cas_rn=465-02-1 | |
Description | CAS Common Chemistry is an open community resource for accessing chemical information. Nearly 500,000 chemical substances from CAS REGISTRY cover areas of community interest, including common and frequently regulated chemicals, and those relevant to high school and undergraduate chemistry classes. This chemical information, curated by our expert scientists, is provided in alignment with our mission as a division of the American Chemical Society. | |
Explanation | The data from CAS Common Chemistry is provided under a CC-BY-NC 4.0 license, unless otherwise stated. | |
Record name | Germanicol | |
Source | ChemIDplus | |
URL | https://pubchem.ncbi.nlm.nih.gov/substance/?source=chemidplus&sourceid=0000465021 | |
Description | ChemIDplus is a free, web search system that provides access to the structure and nomenclature authority files used for the identification of chemical substances cited in National Library of Medicine (NLM) databases, including the TOXNET system. | |
Record name | Olean-18-en-3-ol | |
Source | EPA DSSTox | |
URL | https://comptox.epa.gov/dashboard/DTXSID00963585 | |
Description | DSSTox provides a high quality public chemistry resource for supporting improved predictive toxicology. | |
Record name | GERMANICOL | |
Source | FDA Global Substance Registration System (GSRS) | |
URL | https://gsrs.ncats.nih.gov/ginas/app/beta/substances/7NEX9512DZ | |
Description | The FDA Global Substance Registration System (GSRS) enables the efficient and accurate exchange of information on what substances are in regulated products. Instead of relying on names, which vary across regulatory domains, countries, and regions, the GSRS knowledge base makes it possible for substances to be defined by standardized, scientific descriptions. | |
Explanation | Unless otherwise noted, the contents of the FDA website (www.fda.gov), both text and graphics, are not copyrighted. They are in the public domain and may be republished, reprinted and otherwise used freely by anyone without the need to obtain permission from FDA. Credit to the U.S. Food and Drug Administration as the source is appreciated but not required. | |
Retrosynthesis Analysis
AI-Powered Synthesis Planning: Our tool employs the Template_relevance Pistachio, Template_relevance Bkms_metabolic, Template_relevance Pistachio_ringbreaker, Template_relevance Reaxys, Template_relevance Reaxys_biocatalysis model, leveraging a vast database of chemical reactions to predict feasible synthetic routes.
One-Step Synthesis Focus: Specifically designed for one-step synthesis, it provides concise and direct routes for your target compounds, streamlining the synthesis process.
Accurate Predictions: Utilizing the extensive PISTACHIO, BKMS_METABOLIC, PISTACHIO_RINGBREAKER, REAXYS, REAXYS_BIOCATALYSIS database, our tool offers high-accuracy predictions, reflecting the latest in chemical research and data.
Strategy Settings
Precursor scoring | Relevance Heuristic |
---|---|
Min. plausibility | 0.01 |
Model | Template_relevance |
Template Set | Pistachio/Bkms_metabolic/Pistachio_ringbreaker/Reaxys/Reaxys_biocatalysis |
Top-N result to add to graph | 6 |
Feasible Synthetic Routes
ANone: Germanicol has a molecular formula of C30H50O and a molecular weight of 426.72 g/mol.
A: The structure of this compound has been elucidated through extensive spectroscopic analyses including 1D and 2D NMR spectroscopy (1H and 13C-NMR, DEPTQ, 1H-1H-COSY, NOESY, HSQC, and HMBC), low (GC-MS) and high resolution mass spectrometry (HR-ESI-MS), and infrared (IR) spectroscopy. []
A: this compound is a pentacyclic triterpene found in various plant species. It has been isolated from sources like Barringtonia asiatica leaves [], Ficus species leaves [], Sonchus asper herbs [], Elateriospermum tapos leaves and wood [, ], Scorzonera veratrifolia roots [], Manilkara zapota leaves [], Marsdenia tenacissima stems [], Kleinia odora aerial parts [], Crepis senecioides flowers [], Santalum album leaves [], Bauhinia forficata [], Phoradendron reichenbachianum aerial parts [], Avicennia alba leaves and roots [], Viburnum grandiflorum [], Flindersia bourjotiana leaves [], Anthurus muellerianus spores [], Araujia sericifera aerial parts [], Onoseris salicifolia [], Bejaria resinosa leaves, flowers and fruits [], and Psammosilene tunicoides [].
A: this compound biosynthesis involves oxidosqualene cyclases (OSCs), enzymes that catalyze the cyclization of 2,3-oxidosqualene. In apple fruit, MdOSC4 is a multifunctional OSC that produces this compound, along with β-amyrin and lupeol. [] In Bauhinia forficata, BfOSC2 is the specific OSC responsible for this compound production. [] Research on Euphorbia pulcherrima suggests a dual mechanism for latex triterpene synthesis, including this compound, involving both direct synthesis from acetate and mevalonate in freshly tapped latex and synthesis from sugars or acetic acid in intact plants. []
A: this compound has demonstrated various biological activities, including: * Anticancer activity: this compound induces apoptosis, cell cycle arrest, and inhibits cell migration in human colon cancer cells (HCT-116 and HT29). [] * Antimicrobial activity: It exhibits antifungal activity against Candida albicans and antibacterial activity against Staphylococcus aureus and Pseudomonas aeruginosa. [] * Anti-inflammatory activity: this compound acetate, a derivative of this compound, exhibited significant inhibition of nitrite concentration in LPS-stimulated macrophages, indicating anti-inflammatory potential. []
A: The diverse biological activities of this compound make it a promising candidate for various applications, including: * Development of anticancer agents: Its selective cytotoxicity against colon cancer cells, while showing lower toxicity towards normal colon cells, makes it a potential target for anticancer drug development. [] * Antimicrobial therapies: Its activity against various bacterial and fungal species suggests potential for development of novel antimicrobial agents, particularly against drug-resistant strains. [, ] * Anti-inflammatory drug development: The anti-inflammatory potential of this compound and its derivatives warrants further investigation for the development of new anti-inflammatory therapies. []
A: Various analytical methods are employed for the characterization and quantification of this compound, including: * Gas Chromatography-Mass Spectrometry (GC-MS): This technique is commonly used to identify and quantify this compound in complex mixtures, such as plant extracts and environmental samples. [, , , ] * Nuclear Magnetic Resonance (NMR) spectroscopy: NMR, particularly 1D and 2D techniques, is crucial for determining the structure and stereochemistry of this compound. [, , , ] * High-Performance Thin Layer Chromatography (HPTLC): HPTLC is a rapid and sensitive method for the separation and quantification of this compound in plant materials. []
Avertissement et informations sur les produits de recherche in vitro
Veuillez noter que tous les articles et informations sur les produits présentés sur BenchChem sont destinés uniquement à des fins informatives. Les produits disponibles à l'achat sur BenchChem sont spécifiquement conçus pour des études in vitro, qui sont réalisées en dehors des organismes vivants. Les études in vitro, dérivées du terme latin "in verre", impliquent des expériences réalisées dans des environnements de laboratoire contrôlés à l'aide de cellules ou de tissus. Il est important de noter que ces produits ne sont pas classés comme médicaments et n'ont pas reçu l'approbation de la FDA pour la prévention, le traitement ou la guérison de toute condition médicale, affection ou maladie. Nous devons souligner que toute forme d'introduction corporelle de ces produits chez les humains ou les animaux est strictement interdite par la loi. Il est essentiel de respecter ces directives pour assurer la conformité aux normes légales et éthiques en matière de recherche et d'expérimentation.