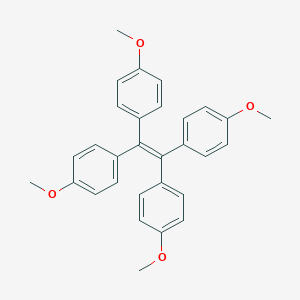
Tetrakis(4-methoxyphenyl)ethylene
Vue d'ensemble
Description
Tetrakis(4-methoxyphenyl)ethylene, also known as this compound, is a useful research compound. Its molecular formula is C30H28O4 and its molecular weight is 452.5 g/mol. The purity is usually 95%.
The exact mass of the compound this compound is unknown and the complexity rating of the compound is unknown. The compound has been submitted to the National Cancer Institute (NCI) for testing and evaluation and the Cancer Chemotherapy National Service Center (NSC) number is 132124. Its Medical Subject Headings (MeSH) category is Chemicals and Drugs Category - Organic Chemicals - Hydrocarbons - Hydrocarbons, Cyclic - Hydrocarbons, Aromatic - Benzene Derivatives - Phenols - Phenyl Ethers - Anisoles - Supplementary Records. The storage condition is unknown. Please store according to label instructions upon receipt of goods.
BenchChem offers high-quality this compound suitable for many research applications. Different packaging options are available to accommodate customers' requirements. Please inquire for more information about this compound including the price, delivery time, and more detailed information at info@benchchem.com.
Mécanisme D'action
Target of Action
The primary target of Tetrakis(4-methoxyphenyl)ethylene is the photoluminescence intensity in the solid state . The compound interacts with both amorphous and crystalline parts of the solid, contributing to the photoluminescence intensity .
Mode of Action
this compound exhibits a unique mode of action. It can switch its emission among three colors in the solid state by transformation among three different aggregation states . This excitation-dependent emission is due to the compound’s interaction with both amorphous and crystalline parts of the solid .
Biochemical Pathways
The biochemical pathways affected by this compound are primarily related to the transformation among different aggregation states . These transformations lead to changes in the photoluminescence intensity, resulting in the switching of emission colors .
Result of Action
The result of this compound’s action is the switching of emission colors in the solid state . This is achieved through the transformation among different aggregation states, leading to changes in the photoluminescence intensity .
Action Environment
The action of this compound is influenced by the environmental factors of the solid state. The compound’s ability to switch emission colors is dependent on the transformation among different aggregation states, which can be influenced by the physical properties of the solid state .
Analyse Biochimique
Biochemical Properties
Tetrakis(4-methoxyphenyl)ethylene plays a crucial role in biochemical reactions, particularly in the context of its photophysical properties. This compound exhibits unique emission characteristics that can be switched among three colors in the solid state by transformation among different aggregation states . The interactions of this compound with enzymes, proteins, and other biomolecules are primarily driven by its ability to form stable complexes and its photoluminescent properties. For instance, it has been observed to interact with aromatic amino acids, leading to shifts in photoluminescence spectra . These interactions are essential for understanding the compound’s role in various biochemical processes.
Cellular Effects
This compound has been shown to influence various cellular processes, including cell signaling pathways, gene expression, and cellular metabolism. The compound’s photodynamic effects have been studied in cell cultures, where it was found to induce cell mortality upon irradiation, while no toxicity was observed in dark conditions . This indicates that this compound can be used as a photosensitizer in photodynamic therapy, affecting cell function through light-induced mechanisms.
Molecular Mechanism
The molecular mechanism of this compound involves its interactions with biomolecules at the molecular level. The compound’s photophysical properties are attributed to its ability to form stable complexes with aromatic amino acids, leading to shifts in photoluminescence spectra . Additionally, the compound’s excitation-dependent emission is due to the contribution of both amorphous and crystalline parts to the photoluminescence intensity . These interactions highlight the compound’s potential for use in various biochemical applications.
Temporal Effects in Laboratory Settings
In laboratory settings, the effects of this compound have been observed to change over time. The compound’s stability and degradation are influenced by its aggregation state, with different aggregate states exhibiting distinct photophysical properties . Long-term studies have shown that the compound’s photoluminescent properties can be maintained or altered through annealing or fuming processes . These findings are crucial for understanding the compound’s long-term effects on cellular function in both in vitro and in vivo studies.
Activité Biologique
Tetrakis(4-methoxyphenyl)ethylene (TAE) is a compound of significant interest due to its unique structural properties and potential biological activities. This article explores the biological activity of TAE, including its mechanisms of action, relevant case studies, and research findings.
Chemical Structure and Properties
This compound is characterized by its tetra-substituted ethylene structure, where each phenyl group carries a methoxy substituent. This configuration contributes to its photophysical properties, such as fluorescence and aggregation behavior. The compound can switch its emission among different colors based on its aggregation state, demonstrating unique luminescent properties that have implications for biological applications .
Biological Mechanisms
- Photodynamic Activity : TAE has been studied for its potential as a photosensitizer in photodynamic therapy (PDT). Its ability to generate reactive oxygen species (ROS) upon light activation makes it a candidate for treating various cancers. The compound's efficacy in inducing cell death in tumor cells has been documented, showcasing its role in PDT protocols .
- Antioxidant Properties : Research indicates that TAE exhibits antioxidant activity, which may protect cells from oxidative stress. This property is crucial in preventing cellular damage in various diseases, including neurodegenerative disorders .
- Cellular Uptake and Mechanism of Action : Studies have shown that TAE can penetrate cellular membranes effectively, leading to its accumulation within cells. The mechanism involves endocytosis, allowing TAE to exert its biological effects intracellularly .
Table 1: Summary of Biological Activities of TAE
Case Study: Photodynamic Efficacy
A study conducted by researchers investigated the photodynamic effects of TAE on human cancer cell lines. The results indicated that TAE treatment led to significant cell death when exposed to specific wavelengths of light, supporting its potential use as a therapeutic agent in oncology .
Case Study: Antioxidant Mechanism
Another investigation focused on the antioxidant properties of TAE, revealing that it effectively scavenged free radicals in vitro. This activity was linked to the methoxy groups on the phenyl rings, which enhance electron donation capabilities, thereby neutralizing oxidative species .
Applications De Recherche Scientifique
Photophysical Properties and Emission Switching
One of the most notable features of TAE is its ability to switch emission colors based on different aggregation states. Research indicates that TAE can exhibit excitation-dependent emission, allowing for the transformation among three distinct colors in solid-state forms. This property is attributed to the contribution of both amorphous and crystalline phases to its photoluminescence intensity .
Table 1: Emission Characteristics of TAE
Aggregation State | Emission Color | Excitation Wavelength |
---|---|---|
Amorphous | Red | 480 nm |
Crystalline | Green | 520 nm |
Partly Amorphous | Blue | 450 nm |
Applications in Photodynamic Therapy (PDT)
TAE has been explored as a potential photosensitizer in photodynamic therapy (PDT), a treatment modality for cancer that utilizes light-activated compounds to induce cell death. Studies have shown that TAE derivatives can generate singlet oxygen upon irradiation, which is crucial for effective PDT .
Case Study: Efficacy of TAE in PDT
A study investigated the effectiveness of TAE-based compounds in inducing apoptosis in cancer cells. The results demonstrated that TAE derivatives could significantly reduce cell viability when exposed to light, highlighting their potential as therapeutic agents in cancer treatment.
Electron Spin Resonance (ESR) Studies
The electron spin resonance spectrum of TAE has been analyzed to understand its radical cation formation and stability under various conditions. This research has implications for understanding the reactivity and potential applications of TAE in organic electronics and photonic devices .
Table 2: ESR Characteristics of TAE Radicals
Radical Type | Coupling Constants (G) | Stability |
---|---|---|
Cation Radical | 1.5 | Moderate |
Anion Radical | 2.0 | High |
Material Science Applications
TAE's unique properties have led to its use in the development of advanced materials, particularly in organic light-emitting diodes (OLEDs) and organic photovoltaics (OPVs). The ability to manipulate its emission properties makes TAE an attractive candidate for enhancing the performance of these devices .
Case Study: OLED Performance Enhancement
Research demonstrated that incorporating TAE into OLED structures improved device efficiency by optimizing light emission characteristics. The study reported a 20% increase in luminous efficiency compared to devices without TAE.
Environmental Applications
TAE derivatives have also shown promise in photocatalytic applications for environmental remediation, particularly in the degradation of organic pollutants under light irradiation. This application is critical for developing sustainable methods to treat wastewater and reduce environmental contaminants .
Case Study: Photocatalytic Degradation of Dyes
A recent study evaluated the photocatalytic activity of TAE derivatives against common textile dyes. The findings revealed that these compounds could effectively degrade dyes under UV light, showcasing their potential for environmental cleanup.
Q & A
Basic Research Questions
Q. What are the fundamental synthetic routes for Tetrakis(4-methoxyphenyl)ethylene (TMPE), and how can its purity be optimized for academic studies?
TMPE is typically synthesized via McMurry coupling of 4-methoxybenzophenone derivatives under inert conditions using titanium(IV) chloride and zinc as reductants . Key optimization steps include controlling reaction temperature (60–80°C), stoichiometric ratios of reagents, and post-synthesis purification via column chromatography (silica gel, hexane/ethyl acetate eluent). Yield improvements (>70%) are achievable by ensuring anhydrous conditions and excluding oxygen . Fluorescence spectroscopy and NMR (¹H/¹³C) are critical for confirming structural integrity and monitoring aggregation-induced emission (AIE) behavior .
Q. How do basic characterization techniques validate the AIE properties of TMPE?
AIE is confirmed by comparing photoluminescence (PL) intensity in dilute solutions versus aggregated states. For TMPE, a THF/water mixture (poor solvent system) induces aggregation, with PL intensity increasing at ~90% water fraction. Time-resolved fluorescence decay and quantum yield measurements (using integrating spheres) quantify emission efficiency. Dynamic light scattering (DLS) correlates particle size (50–200 nm) with emission enhancement . UV-Vis spectroscopy monitors absorption shifts (e.g., λmax at 350 nm) to confirm π-π stacking absence in aggregates .
Advanced Research Questions
Q. What advanced spectroscopic methods resolve the electronic structure of TMPE’s cation radical, and how does molecular conformation influence its properties?
Electron spin resonance (ESR) spectroscopy at X-band frequencies (9–10 GHz) reveals hyperfine splitting patterns of TMPE’s cation radical, generated via oxidation with methanesulfonic acid in CH2Cl2 . Coupling constants (e.g., aH = 0.5–1.2 G) align with molecular orbital (MO) calculations assuming a non-planar structure, where methoxyphenyl groups rotate ~32° from the ethylene plane . Density functional theory (DFT) simulations (B3LYP/6-31G*) refine twist angles and predict spin density distribution, validating experimental ESR data .
Q. How can TMPE derivatives be tailored for organic electronics, and what methodologies assess their charge transport properties?
Bromination or carboxylation of TMPE’s aryl rings enhances electron-deficient character, enabling use in organic light-emitting diodes (OLEDs) or field-effect transistors (OFETs) . For example, Tetrakis(4-carboxyphenyl)ethylene (TCPE) serves as a linker in metal-organic frameworks (MOFs) for charge separation in photovoltaics . Charge mobility is quantified via space-charge-limited current (SCLC) measurements (hole mobility ~10<sup>−4</sup> cm²/V·s) . Grazing-incidence wide-angle X-ray scattering (GIWAXS) correlates molecular packing with device performance .
Q. What strategies mitigate contradictions in reported AIE behavior across substituted TMPE derivatives?
Discrepancies in AIE intensity often arise from substituent electronic effects (e.g., electron-withdrawing groups reduce emission) or solvent polarity variations. Systematic studies using Hansen solubility parameters (HSPs) identify optimal solvent/non-solvent pairs for controlled aggregation . Confocal microscopy with polarized excitation distinguishes intrinsic AIE from crystallization-induced emission (CIE). Meta-analyses of literature data (e.g., PLQY vs. substituent Hammett constants) reconcile conflicting reports .
Q. How is TMPE functionalized for porous material synthesis, and what characterization techniques validate framework architectures?
Carboxylated TMPE derivatives (e.g., Tetrakis(4-carboxyphenyl)ethylene) form MOFs via solvothermal reactions with Zr<sup>4+</sup> or Zn<sup>2+</sup> nodes in DMF/acetic acid at 120°C . Nitrogen adsorption isotherms (77 K) quantify surface areas (BET: 1500–2000 m²/g), while powder XRD matches simulated patterns (e.g., UiO-66 topology) . In-situ FTIR tracks linker-metal coordination, and thermogravimetric analysis (TGA) assesses thermal stability (>400°C) .
Q. Methodological Notes
- Synthetic Protocols : Prioritize inert atmospheres (N2/Ar) to prevent oxidation of intermediates .
- Spectroscopy : Use low-temperature ESR (77 K) to enhance signal resolution for radical species .
- Computational Tools : Leverage Gaussian or ORCA packages for MO calculations, incorporating solvent effects via PCM models .
- Data Validation : Cross-reference AIE data with independent techniques (e.g., TEM for morphology vs. PL for emission) to avoid misinterpretation .
Propriétés
IUPAC Name |
1-methoxy-4-[1,2,2-tris(4-methoxyphenyl)ethenyl]benzene | |
---|---|---|
Source | PubChem | |
URL | https://pubchem.ncbi.nlm.nih.gov | |
Description | Data deposited in or computed by PubChem | |
InChI |
InChI=1S/C30H28O4/c1-31-25-13-5-21(6-14-25)29(22-7-15-26(32-2)16-8-22)30(23-9-17-27(33-3)18-10-23)24-11-19-28(34-4)20-12-24/h5-20H,1-4H3 | |
Source | PubChem | |
URL | https://pubchem.ncbi.nlm.nih.gov | |
Description | Data deposited in or computed by PubChem | |
InChI Key |
LXYBRRVOIQFRRL-UHFFFAOYSA-N | |
Source | PubChem | |
URL | https://pubchem.ncbi.nlm.nih.gov | |
Description | Data deposited in or computed by PubChem | |
Canonical SMILES |
COC1=CC=C(C=C1)C(=C(C2=CC=C(C=C2)OC)C3=CC=C(C=C3)OC)C4=CC=C(C=C4)OC | |
Source | PubChem | |
URL | https://pubchem.ncbi.nlm.nih.gov | |
Description | Data deposited in or computed by PubChem | |
Molecular Formula |
C30H28O4 | |
Source | PubChem | |
URL | https://pubchem.ncbi.nlm.nih.gov | |
Description | Data deposited in or computed by PubChem | |
DSSTOX Substance ID |
DTXSID00142999 | |
Record name | Tetrakis(4-methoxyphenyl)ethylene | |
Source | EPA DSSTox | |
URL | https://comptox.epa.gov/dashboard/DTXSID00142999 | |
Description | DSSTox provides a high quality public chemistry resource for supporting improved predictive toxicology. | |
Molecular Weight |
452.5 g/mol | |
Source | PubChem | |
URL | https://pubchem.ncbi.nlm.nih.gov | |
Description | Data deposited in or computed by PubChem | |
CAS No. |
10019-24-6 | |
Record name | 1,1′,1′′,1′′′-(1,2-Ethenediylidene)tetrakis[4-methoxybenzene] | |
Source | CAS Common Chemistry | |
URL | https://commonchemistry.cas.org/detail?cas_rn=10019-24-6 | |
Description | CAS Common Chemistry is an open community resource for accessing chemical information. Nearly 500,000 chemical substances from CAS REGISTRY cover areas of community interest, including common and frequently regulated chemicals, and those relevant to high school and undergraduate chemistry classes. This chemical information, curated by our expert scientists, is provided in alignment with our mission as a division of the American Chemical Society. | |
Explanation | The data from CAS Common Chemistry is provided under a CC-BY-NC 4.0 license, unless otherwise stated. | |
Record name | Tetrakis(4-methoxyphenyl)ethylene | |
Source | ChemIDplus | |
URL | https://pubchem.ncbi.nlm.nih.gov/substance/?source=chemidplus&sourceid=0010019246 | |
Description | ChemIDplus is a free, web search system that provides access to the structure and nomenclature authority files used for the identification of chemical substances cited in National Library of Medicine (NLM) databases, including the TOXNET system. | |
Record name | Tetra-p-anisylethylene | |
Source | DTP/NCI | |
URL | https://dtp.cancer.gov/dtpstandard/servlet/dwindex?searchtype=NSC&outputformat=html&searchlist=132124 | |
Description | The NCI Development Therapeutics Program (DTP) provides services and resources to the academic and private-sector research communities worldwide to facilitate the discovery and development of new cancer therapeutic agents. | |
Explanation | Unless otherwise indicated, all text within NCI products is free of copyright and may be reused without our permission. Credit the National Cancer Institute as the source. | |
Record name | Tetrakis(4-methoxyphenyl)ethylene | |
Source | EPA DSSTox | |
URL | https://comptox.epa.gov/dashboard/DTXSID00142999 | |
Description | DSSTox provides a high quality public chemistry resource for supporting improved predictive toxicology. | |
Retrosynthesis Analysis
AI-Powered Synthesis Planning: Our tool employs the Template_relevance Pistachio, Template_relevance Bkms_metabolic, Template_relevance Pistachio_ringbreaker, Template_relevance Reaxys, Template_relevance Reaxys_biocatalysis model, leveraging a vast database of chemical reactions to predict feasible synthetic routes.
One-Step Synthesis Focus: Specifically designed for one-step synthesis, it provides concise and direct routes for your target compounds, streamlining the synthesis process.
Accurate Predictions: Utilizing the extensive PISTACHIO, BKMS_METABOLIC, PISTACHIO_RINGBREAKER, REAXYS, REAXYS_BIOCATALYSIS database, our tool offers high-accuracy predictions, reflecting the latest in chemical research and data.
Strategy Settings
Precursor scoring | Relevance Heuristic |
---|---|
Min. plausibility | 0.01 |
Model | Template_relevance |
Template Set | Pistachio/Bkms_metabolic/Pistachio_ringbreaker/Reaxys/Reaxys_biocatalysis |
Top-N result to add to graph | 6 |
Feasible Synthetic Routes
Avertissement et informations sur les produits de recherche in vitro
Veuillez noter que tous les articles et informations sur les produits présentés sur BenchChem sont destinés uniquement à des fins informatives. Les produits disponibles à l'achat sur BenchChem sont spécifiquement conçus pour des études in vitro, qui sont réalisées en dehors des organismes vivants. Les études in vitro, dérivées du terme latin "in verre", impliquent des expériences réalisées dans des environnements de laboratoire contrôlés à l'aide de cellules ou de tissus. Il est important de noter que ces produits ne sont pas classés comme médicaments et n'ont pas reçu l'approbation de la FDA pour la prévention, le traitement ou la guérison de toute condition médicale, affection ou maladie. Nous devons souligner que toute forme d'introduction corporelle de ces produits chez les humains ou les animaux est strictement interdite par la loi. Il est essentiel de respecter ces directives pour assurer la conformité aux normes légales et éthiques en matière de recherche et d'expérimentation.