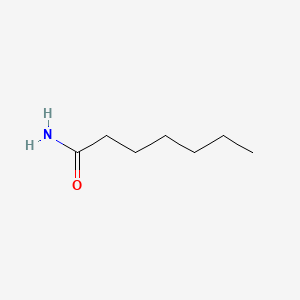
Heptanamide
Vue d'ensemble
Description
Applications De Recherche Scientifique
Heptanamide has several scientific research applications, including:
Mécanisme D'action
Target of Action
Heptanamide, a type of fatty amide , primarily targets the enzyme Limonene-1,2-epoxide hydrolase in Rhodococcus erythropolis . This enzyme plays a crucial role in the metabolism of limonene, a common compound in citrus oils .
Mode of Action
This compound interacts with its target enzyme by serving as a substrate. The enzyme Limonene-1,2-epoxide hydrolase catalyzes the conversion of limonene-1,2-epoxide to limonene-1,2-diol . It can use both the (-) and (+) isomers of limonene-1,2-epoxide as substrates .
Biochemical Pathways
Given its interaction with limonene-1,2-epoxide hydrolase, it’s likely involved in the metabolic pathways of limonene .
Result of Action
The primary result of this compound’s action is the conversion of limonene-1,2-epoxide to limonene-1,2-diol . This conversion is part of the metabolic process of limonene, a compound found in citrus oils .
Analyse Biochimique
Biochemical Properties
Heptanamide plays a significant role in biochemical reactions, particularly in the metabolism of fatty acids. It interacts with various enzymes, proteins, and other biomolecules. One notable interaction is with limonene-1,2-epoxide hydrolase, an enzyme found in Rhodococcus erythropolis . This interaction is crucial for the hydrolysis of epoxides, which are reactive organic compounds. The nature of these interactions involves the binding of this compound to the active site of the enzyme, facilitating the hydrolysis reaction.
Cellular Effects
This compound influences various cellular processes, including cell signaling pathways, gene expression, and cellular metabolism. It has been observed to affect the function of cells by modulating the activity of specific enzymes and proteins involved in metabolic pathways . For instance, this compound’s interaction with limonene-1,2-epoxide hydrolase can impact the detoxification processes within cells, thereby influencing cellular metabolism and overall cell function.
Molecular Mechanism
At the molecular level, this compound exerts its effects through binding interactions with biomolecules. It acts as a substrate for certain enzymes, such as limonene-1,2-epoxide hydrolase, facilitating enzyme-catalyzed reactions . Additionally, this compound can modulate gene expression by influencing transcription factors and other regulatory proteins. This modulation can lead to changes in the expression of genes involved in metabolic pathways and cellular processes.
Temporal Effects in Laboratory Settings
In laboratory settings, the effects of this compound can change over time. Studies have shown that this compound is relatively stable under standard laboratory conditions, but it can degrade over extended periods . The degradation products of this compound may have different biochemical properties and effects on cellular function. Long-term studies have indicated that this compound can have sustained effects on cellular metabolism and gene expression, although these effects may diminish as the compound degrades.
Dosage Effects in Animal Models
The effects of this compound vary with different dosages in animal models. At low doses, this compound has been observed to have minimal toxic effects and can modulate metabolic pathways without causing significant adverse effects . At higher doses, this compound can exhibit toxic effects, including hepatotoxicity and alterations in liver enzyme levels . These threshold effects highlight the importance of dosage regulation in experimental and therapeutic applications.
Metabolic Pathways
This compound is involved in several metabolic pathways, particularly those related to fatty acid metabolism . It interacts with enzymes such as limonene-1,2-epoxide hydrolase, which plays a role in the hydrolysis of epoxides. This interaction can influence metabolic flux and the levels of metabolites within cells. Additionally, this compound can affect the expression of genes involved in metabolic pathways, further modulating cellular metabolism.
Transport and Distribution
Within cells and tissues, this compound is transported and distributed through various mechanisms. It can diffuse across cell membranes due to its lipophilic nature and may interact with transporters and binding proteins that facilitate its movement within the cell . The distribution of this compound within tissues can be influenced by its binding to specific proteins and its solubility in different cellular compartments.
Subcellular Localization
This compound’s subcellular localization is influenced by its chemical properties and interactions with cellular components. It can localize to specific compartments such as the endoplasmic reticulum, mitochondria, and cytoplasm . The targeting of this compound to these compartments can be directed by post-translational modifications and targeting signals that guide its distribution within the cell. This localization can impact its activity and function, as different cellular compartments provide distinct environments for biochemical reactions.
Méthodes De Préparation
L'heptanamide peut être synthétisé par une réaction d'acylation. Une méthode de préparation courante implique la réaction de l'acide heptanoïque avec une amine en présence d'un catalyseur acide dans des conditions appropriées . Cette méthode est largement utilisée en raison de sa simplicité et de son efficacité. Les méthodes de production industrielle peuvent impliquer des voies de synthèse similaires mais à plus grande échelle, garantissant la pureté et le rendement du composé.
Analyse Des Réactions Chimiques
L'heptanamide subit diverses réactions chimiques, notamment :
Hydrolyse : L'this compound peut être hydrolysé pour produire de l'acide heptanoïque et de l'ammoniac en milieu acide ou basique.
Oxydation : Il peut être oxydé pour former de l'acide heptanoïque en utilisant des oxydants forts.
Réduction : L'this compound peut être réduit en heptanamine en utilisant des réducteurs comme l'hydrure de lithium et d'aluminium.
Substitution : Il peut subir des réactions de substitution où le groupe amide est remplacé par d'autres groupes fonctionnels.
Les réactifs couramment utilisés dans ces réactions comprennent les acides, les bases, les oxydants et les réducteurs. Les principaux produits formés à partir de ces réactions sont l'acide heptanoïque, l'heptanamine et les dérivés substitués de l'this compound.
Applications de la recherche scientifique
L'this compound a plusieurs applications de recherche scientifique, notamment :
Industrie : L'this compound est utilisé dans la production de polymères, de résines et d'autres produits chimiques industriels.
Mécanisme d'action
Le mécanisme d'action de l'this compound implique son interaction avec des cibles moléculaires et des voies spécifiques. Par exemple, il peut agir comme un substrat pour des enzymes comme l'hydrolase de l'époxyde de limonène-1,2, catalysant la conversion de l'époxyde de limonène-1,2 en limonène-1,2-diol
Comparaison Avec Des Composés Similaires
L'heptanamide peut être comparé à d'autres amides gras, tels que :
Hexanamide (C6H13NO) : Structure similaire mais avec un atome de carbone en moins, ce qui entraîne des propriétés physiques et chimiques différentes.
Octanamide (C8H17NO) : Structure similaire mais avec un atome de carbone de plus, ce qui entraîne des variations de solubilité et de réactivité.
Nonanamide (C9H19NO) : Un autre amide gras avec une chaîne carbonée plus longue, affectant ses applications industrielles et biologiques.
Propriétés
IUPAC Name |
heptanamide | |
---|---|---|
Source | PubChem | |
URL | https://pubchem.ncbi.nlm.nih.gov | |
Description | Data deposited in or computed by PubChem | |
InChI |
InChI=1S/C7H15NO/c1-2-3-4-5-6-7(8)9/h2-6H2,1H3,(H2,8,9) | |
Source | PubChem | |
URL | https://pubchem.ncbi.nlm.nih.gov | |
Description | Data deposited in or computed by PubChem | |
InChI Key |
AEDIXYWIVPYNBI-UHFFFAOYSA-N | |
Source | PubChem | |
URL | https://pubchem.ncbi.nlm.nih.gov | |
Description | Data deposited in or computed by PubChem | |
Canonical SMILES |
CCCCCCC(=O)N | |
Source | PubChem | |
URL | https://pubchem.ncbi.nlm.nih.gov | |
Description | Data deposited in or computed by PubChem | |
Molecular Formula |
C7H15NO | |
Source | PubChem | |
URL | https://pubchem.ncbi.nlm.nih.gov | |
Description | Data deposited in or computed by PubChem | |
DSSTOX Substance ID |
DTXSID50211886 | |
Record name | Heptanamide | |
Source | EPA DSSTox | |
URL | https://comptox.epa.gov/dashboard/DTXSID50211886 | |
Description | DSSTox provides a high quality public chemistry resource for supporting improved predictive toxicology. | |
Molecular Weight |
129.20 g/mol | |
Source | PubChem | |
URL | https://pubchem.ncbi.nlm.nih.gov | |
Description | Data deposited in or computed by PubChem | |
CAS No. |
628-62-6 | |
Record name | Heptanamide | |
Source | CAS Common Chemistry | |
URL | https://commonchemistry.cas.org/detail?cas_rn=628-62-6 | |
Description | CAS Common Chemistry is an open community resource for accessing chemical information. Nearly 500,000 chemical substances from CAS REGISTRY cover areas of community interest, including common and frequently regulated chemicals, and those relevant to high school and undergraduate chemistry classes. This chemical information, curated by our expert scientists, is provided in alignment with our mission as a division of the American Chemical Society. | |
Explanation | The data from CAS Common Chemistry is provided under a CC-BY-NC 4.0 license, unless otherwise stated. | |
Record name | Heptanamide | |
Source | ChemIDplus | |
URL | https://pubchem.ncbi.nlm.nih.gov/substance/?source=chemidplus&sourceid=0000628626 | |
Description | ChemIDplus is a free, web search system that provides access to the structure and nomenclature authority files used for the identification of chemical substances cited in National Library of Medicine (NLM) databases, including the TOXNET system. | |
Record name | Heptanamide | |
Source | DrugBank | |
URL | https://www.drugbank.ca/drugs/DB02641 | |
Description | The DrugBank database is a unique bioinformatics and cheminformatics resource that combines detailed drug (i.e. chemical, pharmacological and pharmaceutical) data with comprehensive drug target (i.e. sequence, structure, and pathway) information. | |
Explanation | Creative Common's Attribution-NonCommercial 4.0 International License (http://creativecommons.org/licenses/by-nc/4.0/legalcode) | |
Record name | Heptanamide | |
Source | DTP/NCI | |
URL | https://dtp.cancer.gov/dtpstandard/servlet/dwindex?searchtype=NSC&outputformat=html&searchlist=3819 | |
Description | The NCI Development Therapeutics Program (DTP) provides services and resources to the academic and private-sector research communities worldwide to facilitate the discovery and development of new cancer therapeutic agents. | |
Explanation | Unless otherwise indicated, all text within NCI products is free of copyright and may be reused without our permission. Credit the National Cancer Institute as the source. | |
Record name | Heptanamide | |
Source | EPA DSSTox | |
URL | https://comptox.epa.gov/dashboard/DTXSID50211886 | |
Description | DSSTox provides a high quality public chemistry resource for supporting improved predictive toxicology. | |
Record name | HEPTANAMIDE | |
Source | FDA Global Substance Registration System (GSRS) | |
URL | https://gsrs.ncats.nih.gov/ginas/app/beta/substances/6QX6H3415T | |
Description | The FDA Global Substance Registration System (GSRS) enables the efficient and accurate exchange of information on what substances are in regulated products. Instead of relying on names, which vary across regulatory domains, countries, and regions, the GSRS knowledge base makes it possible for substances to be defined by standardized, scientific descriptions. | |
Explanation | Unless otherwise noted, the contents of the FDA website (www.fda.gov), both text and graphics, are not copyrighted. They are in the public domain and may be republished, reprinted and otherwise used freely by anyone without the need to obtain permission from FDA. Credit to the U.S. Food and Drug Administration as the source is appreciated but not required. | |
Retrosynthesis Analysis
AI-Powered Synthesis Planning: Our tool employs the Template_relevance Pistachio, Template_relevance Bkms_metabolic, Template_relevance Pistachio_ringbreaker, Template_relevance Reaxys, Template_relevance Reaxys_biocatalysis model, leveraging a vast database of chemical reactions to predict feasible synthetic routes.
One-Step Synthesis Focus: Specifically designed for one-step synthesis, it provides concise and direct routes for your target compounds, streamlining the synthesis process.
Accurate Predictions: Utilizing the extensive PISTACHIO, BKMS_METABOLIC, PISTACHIO_RINGBREAKER, REAXYS, REAXYS_BIOCATALYSIS database, our tool offers high-accuracy predictions, reflecting the latest in chemical research and data.
Strategy Settings
Precursor scoring | Relevance Heuristic |
---|---|
Min. plausibility | 0.01 |
Model | Template_relevance |
Template Set | Pistachio/Bkms_metabolic/Pistachio_ringbreaker/Reaxys/Reaxys_biocatalysis |
Top-N result to add to graph | 6 |
Feasible Synthetic Routes
Q1: What is the mechanism of action for Heptanamide derivatives as histone deacetylase (HDAC) inhibitors?
A1: While the provided abstracts do not delve into specific mechanistic details for all this compound derivatives, they highlight the potent HDAC inhibitory activity of certain analogs. For example, Quinazolin-4(3H)-one-based hydroxamic acids incorporating this compound demonstrated sub-micromolar IC50 values for HDAC inhibition. [] Molecular docking simulations suggested that these compounds, particularly N-Hydroxy-7-(7-methyl-4-oxoquinazolin-3(4H)-yl)this compound (5b) and N-Hydroxy-7-(6-methyl-4-oxoquinazolin-3(4H)-yl)this compound (5c), exhibited strong binding affinity to the active site of HDAC2, surpassing that of SAHA (Vorinostat). [] This binding interaction is likely responsible for the observed HDAC inhibition.
Q2: What is the significance of the solid-solid phase transition observed in this compound monolayers on graphite?
A2: this compound exhibits a unique solid-solid phase transition at 330 K from a pgg symmetry to a p2 symmetry in its monolayer form on graphite. [] This transition is unusual among alkylamides of similar chain lengths, suggesting specific intermolecular interactions influenced by the this compound structure. Further research could elucidate the exact nature of these interactions and their implications for potential applications.
Q3: How does the presence of an amide group influence the self-assembled monolayer (SAM) formation of N-(2-mercaptoethyl)this compound (MEHA) on gold surfaces?
A3: Compared to decanethiol (DT) SAMs, MEHA SAMs demonstrate enhanced thermal stability, attributed to the formation of internal hydrogen bond networks facilitated by the amide group. [] This finding highlights the role of specific functional groups in dictating the properties and stability of SAMs.
Q4: Can this compound derivatives act as latency-reversing agents (LRAs) for HIV?
A4: Research suggests that some this compound derivatives show potential as LRAs by inhibiting HDAC activity. Virtual screening identified four this compound derivatives as potential LRAs. [] Subsequent in vitro studies confirmed that these compounds, including (S)-N-Hydroxy-4-(3-methyl-2-phenylbutanamido)benzamide (compound 15), N-(4-Aminophenyl)this compound (16), N-[4-(Heptanoylamino)phenyl]this compound (17), and 4-(1,3-Dioxo-1H-benzo[de]isoquinolin-2(3H)-yl)-N-(2-hydroxyethyl)butanamide (18), displayed either HDAC inhibitory activity or HIV latency reversal capabilities. []
Q5: How do different host plants influence the phytochemical profile of the parasitic plant Cuscuta reflexa?
A5: GC-MS analysis revealed distinct phytochemical variations in Cuscuta reflexa when grown on Cassia fistula compared to Ficus benghalensis. [] For instance, compounds like 1H-1,2,4triazol-5-amine-1-ethyl, D-glucitol-4-O-hexyl, 3,4,5-trimethoxy cinnamic acid, 3,6-dimethoxy phenanthrene, and 3,5-di tert butyl 4-hydroxyanisol were exclusively found in Cuscuta reflexa parasitizing Cassia fistula. [] Conversely, vanillin, 3-aminopyrrolidine, cetene, sarcosine N-isobutyryl-tetra decyl ester, 4-((1E)-3-hydroxy-1-propenyl)-2-methoxyphenol, 1,5-diphenyl-2H-1,2,4 triazoline-3-thione, 1-octadecene, this compound,N-(1cyclohexylethyl)-2-methyl, scoparone, and 3’methyl-2-benzyledine-coumaran-3-one were only detected in Cuscuta reflexa parasitizing Ficus benghalensis. [] These findings suggest the host plant plays a crucial role in shaping the parasite's chemical composition.
Q6: Has this compound been used to create water-repellent coatings on textiles?
A6: Yes, a derivative of this compound, specifically 2,2,3,3,4,4,5,5,6,6,7,7,7-tridecafluoro-N-[3-(triethoxysilyl)propyl]-heptanamide, has been successfully employed for creating protective hydrophobic and oleophobic coatings on various textiles, including cotton, wool, and polyester. [] This compound imparts impressive water repellency to the treated fabric, achieving a water contact angle of at least 120 degrees. []
Avertissement et informations sur les produits de recherche in vitro
Veuillez noter que tous les articles et informations sur les produits présentés sur BenchChem sont destinés uniquement à des fins informatives. Les produits disponibles à l'achat sur BenchChem sont spécifiquement conçus pour des études in vitro, qui sont réalisées en dehors des organismes vivants. Les études in vitro, dérivées du terme latin "in verre", impliquent des expériences réalisées dans des environnements de laboratoire contrôlés à l'aide de cellules ou de tissus. Il est important de noter que ces produits ne sont pas classés comme médicaments et n'ont pas reçu l'approbation de la FDA pour la prévention, le traitement ou la guérison de toute condition médicale, affection ou maladie. Nous devons souligner que toute forme d'introduction corporelle de ces produits chez les humains ou les animaux est strictement interdite par la loi. Il est essentiel de respecter ces directives pour assurer la conformité aux normes légales et éthiques en matière de recherche et d'expérimentation.