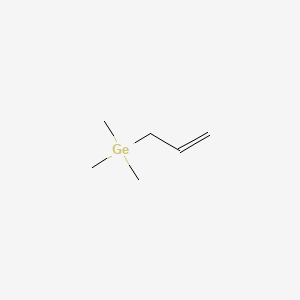
ALLYLTRIMETHYLGERMANE
Vue d'ensemble
Description
ALLYLTRIMETHYLGERMANE is an organogermanium compound with the molecular formula C6H14Ge. It is a colorless liquid that is used in various chemical reactions and industrial applications. The compound is known for its unique properties, which make it valuable in the field of organometallic chemistry.
Méthodes De Préparation
ALLYLTRIMETHYLGERMANE can be synthesized through several methods. One common synthetic route involves the reaction of allyl bromide with trimethylgermanium chloride in the presence of a base such as sodium or potassium. The reaction typically takes place in an organic solvent like diethyl ether or benzene at room temperature. The product is then purified through distillation .
Analyse Des Réactions Chimiques
ALLYLTRIMETHYLGERMANE undergoes various types of chemical reactions, including:
Hydrosilylation: This reaction involves the addition of silicon-hydrogen bonds to the carbon-carbon double bond in this compound.
Oxidation: The compound can be oxidized using oxidizing agents like hydrogen peroxide or ozone, leading to the formation of germanium oxides.
Substitution: this compound can undergo substitution reactions where the allyl group is replaced by other functional groups. This is often achieved using reagents like halogens or organolithium compounds.
Applications De Recherche Scientifique
ALLYLTRIMETHYLGERMANE has several applications in scientific research:
Chemistry: It is used as a precursor in the synthesis of other organogermanium compounds. Its unique reactivity makes it valuable in studying reaction mechanisms and developing new synthetic methodologies.
Biology and Medicine: Research has explored the potential biological activities of organogermanium compounds, including this compound. These studies aim to understand their effects on cellular processes and their potential therapeutic applications.
Mécanisme D'action
The mechanism of action of ALLYLTRIMETHYLGERMANE involves its interaction with various molecular targets. In hydrosilylation reactions, for example, the compound reacts with hydrosilanes in the presence of a catalyst, leading to the formation of new carbon-silicon bonds. The reaction proceeds through the formation of a transition state where the germanium atom coordinates with the catalyst and the silicon-hydrogen bond .
Comparaison Avec Des Composés Similaires
ALLYLTRIMETHYLGERMANE is similar to other organogermanium compounds such as allyltrichlorogermane and allyltrimethylsilane. it is unique in its reactivity and the types of reactions it undergoes. For instance, allyltrichlorogermane has a higher reactivity in hydrosilylation reactions compared to this compound due to the presence of chlorine atoms, which influence the electronic properties of the molecule .
Similar Compounds
- Allyltrichlorogermane
- Allyltrimethylsilane
- Allylmethylchlorosilane
These compounds share similar structural features but differ in their reactivity and applications.
Propriétés
IUPAC Name |
trimethyl(prop-2-enyl)germane | |
---|---|---|
Source | PubChem | |
URL | https://pubchem.ncbi.nlm.nih.gov | |
Description | Data deposited in or computed by PubChem | |
InChI |
InChI=1S/C6H14Ge/c1-5-6-7(2,3)4/h5H,1,6H2,2-4H3 | |
Source | PubChem | |
URL | https://pubchem.ncbi.nlm.nih.gov | |
Description | Data deposited in or computed by PubChem | |
InChI Key |
FBJPYOIWICJHGX-UHFFFAOYSA-N | |
Source | PubChem | |
URL | https://pubchem.ncbi.nlm.nih.gov | |
Description | Data deposited in or computed by PubChem | |
Canonical SMILES |
C[Ge](C)(C)CC=C | |
Source | PubChem | |
URL | https://pubchem.ncbi.nlm.nih.gov | |
Description | Data deposited in or computed by PubChem | |
Molecular Formula |
C6H14Ge | |
Source | PubChem | |
URL | https://pubchem.ncbi.nlm.nih.gov | |
Description | Data deposited in or computed by PubChem | |
DSSTOX Substance ID |
DTXSID40227057 | |
Record name | Germane, trimethyl-2-propenyl- | |
Source | EPA DSSTox | |
URL | https://comptox.epa.gov/dashboard/DTXSID40227057 | |
Description | DSSTox provides a high quality public chemistry resource for supporting improved predictive toxicology. | |
Molecular Weight |
158.81 g/mol | |
Source | PubChem | |
URL | https://pubchem.ncbi.nlm.nih.gov | |
Description | Data deposited in or computed by PubChem | |
CAS No. |
762-66-3 | |
Record name | Germane, trimethyl-2-propenyl- | |
Source | ChemIDplus | |
URL | https://pubchem.ncbi.nlm.nih.gov/substance/?source=chemidplus&sourceid=0000762663 | |
Description | ChemIDplus is a free, web search system that provides access to the structure and nomenclature authority files used for the identification of chemical substances cited in National Library of Medicine (NLM) databases, including the TOXNET system. | |
Record name | Germane, trimethyl-2-propenyl- | |
Source | EPA DSSTox | |
URL | https://comptox.epa.gov/dashboard/DTXSID40227057 | |
Description | DSSTox provides a high quality public chemistry resource for supporting improved predictive toxicology. | |
Retrosynthesis Analysis
AI-Powered Synthesis Planning: Our tool employs the Template_relevance Pistachio, Template_relevance Bkms_metabolic, Template_relevance Pistachio_ringbreaker, Template_relevance Reaxys, Template_relevance Reaxys_biocatalysis model, leveraging a vast database of chemical reactions to predict feasible synthetic routes.
One-Step Synthesis Focus: Specifically designed for one-step synthesis, it provides concise and direct routes for your target compounds, streamlining the synthesis process.
Accurate Predictions: Utilizing the extensive PISTACHIO, BKMS_METABOLIC, PISTACHIO_RINGBREAKER, REAXYS, REAXYS_BIOCATALYSIS database, our tool offers high-accuracy predictions, reflecting the latest in chemical research and data.
Strategy Settings
Precursor scoring | Relevance Heuristic |
---|---|
Min. plausibility | 0.01 |
Model | Template_relevance |
Template Set | Pistachio/Bkms_metabolic/Pistachio_ringbreaker/Reaxys/Reaxys_biocatalysis |
Top-N result to add to graph | 6 |
Feasible Synthetic Routes
Avertissement et informations sur les produits de recherche in vitro
Veuillez noter que tous les articles et informations sur les produits présentés sur BenchChem sont destinés uniquement à des fins informatives. Les produits disponibles à l'achat sur BenchChem sont spécifiquement conçus pour des études in vitro, qui sont réalisées en dehors des organismes vivants. Les études in vitro, dérivées du terme latin "in verre", impliquent des expériences réalisées dans des environnements de laboratoire contrôlés à l'aide de cellules ou de tissus. Il est important de noter que ces produits ne sont pas classés comme médicaments et n'ont pas reçu l'approbation de la FDA pour la prévention, le traitement ou la guérison de toute condition médicale, affection ou maladie. Nous devons souligner que toute forme d'introduction corporelle de ces produits chez les humains ou les animaux est strictement interdite par la loi. Il est essentiel de respecter ces directives pour assurer la conformité aux normes légales et éthiques en matière de recherche et d'expérimentation.