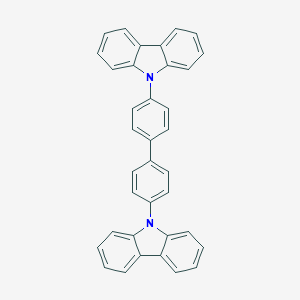
4,4'-Bis(N-carbazolyl)-1,1'-biphenyl
Vue d'ensemble
Description
4,4’-Bis(N-carbazolyl)-1,1’-biphenyl, also known as CBP, is a hole-transport material used in high-efficiency red OLEDs and electroluminescent dendritic complexes .
Molecular Structure Analysis
The molecular structure of 4,4’-Bis(N-carbazolyl)-1,1’-biphenyl is characterized by its electron-rich property from two carbazolyl units . This property makes it one of the most widely-used host materials for efficient fluorescent and phosphorescent organic light-emitting diodes with high hole mobility .Physical And Chemical Properties Analysis
4,4’-Bis(N-carbazolyl)-1,1’-biphenyl has a chemical formula of C36H24N2 and a molecular weight of 484.59 g/mol . It has a melting point of 281-285°C . The HOMO and LUMO energy levels are 6.0 eV and 2.9 eV, respectively .Applications De Recherche Scientifique
Organic Light-Emitting Diodes (OLEDs)
4,4’-Bis(N-carbazolyl)-1,1’-biphenyl, also known as CBP, is widely used as a host material in OLEDs. Its good solubility and film-forming properties make it suitable for creating the emissive layer of OLEDs through cost-effective methods like spin coating from solutions .
High-Efficiency Red OLEDs
CBP serves as a hole-transporting material in high-efficiency red OLEDs and electroluminescent dendritic complexes. Its role is crucial in achieving high luminance and device performance .
Electropolymerization
The compound has been utilized in the synthesis of carbazole derivatives for electropolymerization. This process is significant in creating chiral hybrid sensors for specific applications, such as detecting L-Phenylalanine (L-Phe) through electrochemically crosslinking polymer precursors .
Mécanisme D'action
Target of Action
The primary target of 4,4’-Bis(N-carbazolyl)-1,1’-biphenyl, also known as 4,4’-Di(9H-carbazol-9-yl)-1,1’-biphenyl, is the electron transport chain in organic light-emitting diodes (OLEDs) . The compound is used as a host material in OLEDs , where it plays a crucial role in transporting electrons and holes .
Mode of Action
The compound interacts with its targets by facilitating the injection and transport of electrons . It has been observed that the introduction of phosphonate substitutes can endow the compound with excellent electron injection/transport ability . As a result, the compound shows an electron-dominated behavior observed in single carrier devices .
Biochemical Pathways
The compound affects the electron transport pathway in OLEDs . The electron transport pathway is crucial for the functioning of OLEDs, as it influences the device’s performance, including its luminous efficiency .
Pharmacokinetics
While the term “pharmacokinetics” is typically used in the context of drug metabolism, in the case of this compound, we can discuss its properties related to its distribution and behavior in the OLEDs. The compound’s highest occupied molecular orbital (HOMO) level is reduced, leading to a large hole injection barrier . This property impacts the compound’s distribution and behavior in the device, influencing its overall performance .
Result of Action
The action of the compound results in a significant increase in the peak luminous efficiency of the OLEDs . Specifically, the peak luminous efficiency significantly increases from 1.7 cd A −1 of CBP to 31.4 cd A −1 of PCBP .
Action Environment
The action, efficacy, and stability of the compound can be influenced by various environmental factors. For instance, the deposition of the compound onto cooled substrates can result in smaller agglomerates, yielding a higher efficiency
Propriétés
IUPAC Name |
9-[4-(4-carbazol-9-ylphenyl)phenyl]carbazole | |
---|---|---|
Source | PubChem | |
URL | https://pubchem.ncbi.nlm.nih.gov | |
Description | Data deposited in or computed by PubChem | |
InChI |
InChI=1S/C36H24N2/c1-5-13-33-29(9-1)30-10-2-6-14-34(30)37(33)27-21-17-25(18-22-27)26-19-23-28(24-20-26)38-35-15-7-3-11-31(35)32-12-4-8-16-36(32)38/h1-24H | |
Source | PubChem | |
URL | https://pubchem.ncbi.nlm.nih.gov | |
Description | Data deposited in or computed by PubChem | |
InChI Key |
VFUDMQLBKNMONU-UHFFFAOYSA-N | |
Source | PubChem | |
URL | https://pubchem.ncbi.nlm.nih.gov | |
Description | Data deposited in or computed by PubChem | |
Canonical SMILES |
C1=CC=C2C(=C1)C3=CC=CC=C3N2C4=CC=C(C=C4)C5=CC=C(C=C5)N6C7=CC=CC=C7C8=CC=CC=C86 | |
Source | PubChem | |
URL | https://pubchem.ncbi.nlm.nih.gov | |
Description | Data deposited in or computed by PubChem | |
Molecular Formula |
C36H24N2 | |
Source | PubChem | |
URL | https://pubchem.ncbi.nlm.nih.gov | |
Description | Data deposited in or computed by PubChem | |
DSSTOX Substance ID |
DTXSID30460032 | |
Record name | 9,9'-([1,1'-Biphenyl]-4,4'-diyl)di(9H-carbazole) | |
Source | EPA DSSTox | |
URL | https://comptox.epa.gov/dashboard/DTXSID30460032 | |
Description | DSSTox provides a high quality public chemistry resource for supporting improved predictive toxicology. | |
Molecular Weight |
484.6 g/mol | |
Source | PubChem | |
URL | https://pubchem.ncbi.nlm.nih.gov | |
Description | Data deposited in or computed by PubChem | |
Product Name |
4,4'-Bis(N-carbazolyl)-1,1'-biphenyl | |
CAS RN |
58328-31-7 | |
Record name | 9,9'-([1,1'-Biphenyl]-4,4'-diyl)di(9H-carbazole) | |
Source | EPA DSSTox | |
URL | https://comptox.epa.gov/dashboard/DTXSID30460032 | |
Description | DSSTox provides a high quality public chemistry resource for supporting improved predictive toxicology. | |
Record name | 4,4'-Bis(9H-carbazol-9-yl)biphenyl | |
Source | European Chemicals Agency (ECHA) | |
URL | https://echa.europa.eu/information-on-chemicals | |
Description | The European Chemicals Agency (ECHA) is an agency of the European Union which is the driving force among regulatory authorities in implementing the EU's groundbreaking chemicals legislation for the benefit of human health and the environment as well as for innovation and competitiveness. | |
Explanation | Use of the information, documents and data from the ECHA website is subject to the terms and conditions of this Legal Notice, and subject to other binding limitations provided for under applicable law, the information, documents and data made available on the ECHA website may be reproduced, distributed and/or used, totally or in part, for non-commercial purposes provided that ECHA is acknowledged as the source: "Source: European Chemicals Agency, http://echa.europa.eu/". Such acknowledgement must be included in each copy of the material. ECHA permits and encourages organisations and individuals to create links to the ECHA website under the following cumulative conditions: Links can only be made to webpages that provide a link to the Legal Notice page. | |
Synthesis routes and methods I
Procedure details
Synthesis routes and methods II
Procedure details
Synthesis routes and methods III
Procedure details
Synthesis routes and methods IV
Procedure details
Retrosynthesis Analysis
AI-Powered Synthesis Planning: Our tool employs the Template_relevance Pistachio, Template_relevance Bkms_metabolic, Template_relevance Pistachio_ringbreaker, Template_relevance Reaxys, Template_relevance Reaxys_biocatalysis model, leveraging a vast database of chemical reactions to predict feasible synthetic routes.
One-Step Synthesis Focus: Specifically designed for one-step synthesis, it provides concise and direct routes for your target compounds, streamlining the synthesis process.
Accurate Predictions: Utilizing the extensive PISTACHIO, BKMS_METABOLIC, PISTACHIO_RINGBREAKER, REAXYS, REAXYS_BIOCATALYSIS database, our tool offers high-accuracy predictions, reflecting the latest in chemical research and data.
Strategy Settings
Precursor scoring | Relevance Heuristic |
---|---|
Min. plausibility | 0.01 |
Model | Template_relevance |
Template Set | Pistachio/Bkms_metabolic/Pistachio_ringbreaker/Reaxys/Reaxys_biocatalysis |
Top-N result to add to graph | 6 |
Feasible Synthetic Routes
Q & A
Q1: What is the molecular formula and weight of CBP?
A1: CBP has a molecular formula of C36H24N2 and a molecular weight of 492.60 g/mol.
Q2: What is the glass transition temperature (Tg) of CBP?
A2: The glass transition temperature of CBP is relatively high, measured at 151 °C. [] This thermal stability makes CBP suitable for use in OLED devices that can reach high operating temperatures.
Q3: How does the molecular structure of CBP influence its film formation and morphology?
A3: Molecular dynamics simulations of CBP in chloroform solution reveal that during solvent evaporation, solute aggregation initiates at the solution-vapor interface, leading to non-homogeneous solvent distribution within the film. [] This non-uniformity can induce preferential alignment of host molecules and likely results in some solvent remaining trapped within the film.
Q4: Can the orientation of CBP molecules within a thin film be controlled?
A4: Yes, studies using vibrational sum frequency generation (VSFG) spectroscopy show that the orientation of CBP molecules at interfaces can be influenced by the substrate. [] For instance, CBP molecules tend to orient more vertically (edge-on) at a buried CaF2 interface compared to a free air interface, likely due to intermolecular π-π stacking interactions.
Q5: Does the deposition temperature affect the orientation of CBP molecules in thin films?
A5: Yes, studies have shown that CBP molecules adopt random orientations in films fabricated at higher temperatures (350 K). [] This random orientation leads to lower hole mobility in the film. Conversely, lower deposition temperatures can promote a more horizontal orientation of CBP molecules.
Q6: What is the primary role of CBP in OLEDs?
A6: CBP is commonly employed as a host material in the emissive layer of OLEDs, particularly for phosphorescent and thermally activated delayed fluorescence (TADF) devices. [, ]
Q7: Why is CBP considered a suitable host material for phosphorescent OLEDs?
A7: CBP possesses suitable energy levels and triplet energy that allow for efficient energy transfer to phosphorescent dopants, resulting in efficient light emission. [] This property has been demonstrated in devices utilizing red phosphorescent iridium(III) complexes doped into CBP.
Q8: Can CBP be used in solution-processed OLEDs?
A9: Yes, CBP is soluble in common organic solvents, enabling its use in solution-processing techniques for OLED fabrication. [, ] This is advantageous for large-area and flexible OLED applications.
Q9: How does the deposition rate of CBP affect the performance of OLEDs?
A10: Research indicates that while high deposition rates of CBP (up to 50 Å/s) do not lead to gas-phase nucleation, they can cause increased efficiency roll-off at high currents due to enhanced triplet-polaron annihilation. [] This effect is attributed to stress accumulation within the CBP film, generating defect states that act as recombination sites.
Q10: How does CBP compare to other host materials in terms of its impact on the efficiency roll-off in TADF-based OLEDs?
A12: Studies have shown that the molecular orientation of the host material can significantly influence the efficiency roll-off characteristics in TADF OLEDs. For instance, randomly oriented CBP molecules, achieved by fabrication at high temperatures, led to a 30% suppression of efficiency roll-off at high current densities compared to more ordered CBP films. []
Q11: What is the role of CBP in hybrid charge transfer exciton (HCTE) confinement?
A14: Research shows that in organic-inorganic quantum wells (QWs) utilizing CBP, the confinement of HCTEs is less efficient compared to QWs using tetraphenyldibenzoperiflanthene (DBP). [] This difference is attributed to the lower energy of the highest occupied molecular orbital (HOMO) level in CBP compared to DBP.
Q12: How do metal nanoparticles influence the performance of CBP-based OLEDs?
A15: Studies on blue micro-OLEDs incorporating aluminum nanoparticle arrays on the anode revealed a suppression of exciplex emission at the NPB/CBP interface. [] Additionally, the localized surface plasmon resonance (LSPR) of the nanoparticles enhanced CBP emission due to energy coupling, ultimately improving device efficiency.
Q13: How does the presence of ZnCl2 affect the photoluminescence of CBP derivatives?
A16: Interestingly, certain CBP derivatives exhibit a strong and selective spectral response to ZnCl2. [] This property makes them potential candidates for developing optical sensors for this specific analyte.
Q14: How does CBP affect the stability of OLED devices?
A17: The high glass transition temperature of CBP contributes to improved thermal stability of OLED devices, allowing for operation at higher temperatures without significant degradation. []
Q15: How does CBP influence the charge balance in OLEDs?
A19: Studies on green phosphorescent OLEDs employing CBP in mixed host emission layers (MH-EMLs) have demonstrated improved charge balance compared to single-host devices. [] This is attributed to the confinement of holes in the EML due to the deep HOMO level of CBP, facilitating more efficient exciton formation and a wider recombination zone.
Q16: How is computational chemistry used to study CBP and its derivatives?
A20: Computational methods like density functional theory (DFT) are employed to investigate the electronic structure, optical properties, and charge transport characteristics of CBP and its derivatives. [] These calculations aid in understanding the relationship between molecular structure and material properties, guiding the design of new and improved OLED materials.
Q17: Are there specific force field parameters tailored for simulating CBP in solid-state simulations?
A21: Yes, researchers have developed united atom force field parameters specifically optimized for simulating CBP and other OLED materials in the solid phase. [] This force field accurately reproduces experimental crystal structures and melting temperatures, making it valuable for studying the morphology of emissive layers in OLED devices.
Avertissement et informations sur les produits de recherche in vitro
Veuillez noter que tous les articles et informations sur les produits présentés sur BenchChem sont destinés uniquement à des fins informatives. Les produits disponibles à l'achat sur BenchChem sont spécifiquement conçus pour des études in vitro, qui sont réalisées en dehors des organismes vivants. Les études in vitro, dérivées du terme latin "in verre", impliquent des expériences réalisées dans des environnements de laboratoire contrôlés à l'aide de cellules ou de tissus. Il est important de noter que ces produits ne sont pas classés comme médicaments et n'ont pas reçu l'approbation de la FDA pour la prévention, le traitement ou la guérison de toute condition médicale, affection ou maladie. Nous devons souligner que toute forme d'introduction corporelle de ces produits chez les humains ou les animaux est strictement interdite par la loi. Il est essentiel de respecter ces directives pour assurer la conformité aux normes légales et éthiques en matière de recherche et d'expérimentation.