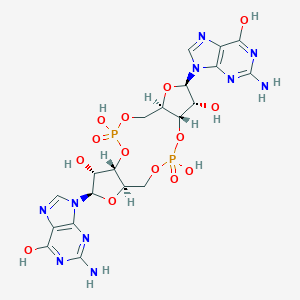
c-di-GMP
Vue d'ensemble
Description
Le bis-(3’, 5’)-diguanosine monophosphate cyclique (disodique) est une molécule de signalisation à base de nucléotides découverte en 1987. C’est un second messager qui joue un rôle crucial dans la régulation de divers processus biologiques chez les bactéries, notamment la motilité, la formation de biofilms, la virulence et la progression du cycle cellulaire . Ce composé est également connu pour sa capacité à se lier à des récepteurs spécifiques dans les cellules humaines, modulant les réponses cellulaires de l’hôte et présentant un potentiel en tant que cible thérapeutique .
Applications De Recherche Scientifique
Mécanisme D'action
Le bis-(3’, 5’)-diguanosine monophosphate cyclique (disodique) exerce ses effets en se liant à des récepteurs spécifiques dans les cellules bactériennes et eucaryotes. Chez les bactéries, il régule diverses activités cellulaires en interagissant avec les diguanylate cyclases et les phosphodiestérases, qui contrôlent sa concentration intracellulaire . Dans les cellules eucaryotes, il se lie à des récepteurs tels que le STING (stimulateur des gènes de l’interféron), modulant les réponses immunitaires et présentant des propriétés anticancéreuses .
Analyse Biochimique
Biochemical Properties
Cyclic Diguanylic Acid interacts with a wide range of enzymes, proteins, and other biomolecules. The intracellular concentration of Cyclic Diguanylic Acid is controlled by diguanylate cyclases (DGCs) and phosphodiesterases (PDEs) . DGCs catalyze Cyclic Diguanylic Acid production by consuming two GTP molecules, whereas PDEs catalyze the degradation of Cyclic Diguanylic Acid into a pGpG linear nucleotide or two GTP molecules . Signal transduction occurs through Cyclic Diguanylic Acid binding to downstream receptors, triggered by sensing environmental or cellular cues .
Cellular Effects
Cyclic Diguanylic Acid has a profound impact on various types of cells and cellular processes. It influences cell function by regulating cell motility, biofilm formation and dispersion, cell division, differentiation, quorum sensing, and virulence . It also controls transcription initiation and termination, translation, exopolysaccharide (EPS) synthesis and secretion, flagellar and type III secretion system assembly, cell cycle progression, proteolysis of target proteins, and involvement in the localization of target proteins .
Molecular Mechanism
Cyclic Diguanylic Acid exerts its effects at the molecular level through binding interactions with biomolecules, enzyme inhibition or activation, and changes in gene expression . It binds to a diverse array of protein or RNA effectors which include PilZ domain-containing proteins, transcription factors, proteases, kinases, and riboswitches .
Temporal Effects in Laboratory Settings
In laboratory settings, Cyclic Diguanylic Acid shows dynamic changes with high temporal resolution . A genetically encoded biosensor has been developed to track Cyclic Diguanylic Acid in single cells and with high temporal resolution over extended imaging times .
Dosage Effects in Animal Models
In animal models, the effects of Cyclic Diguanylic Acid vary with different dosages . It enhances protective innate immunity in a murine model of pertussis . The immune responses were associated with an earlier and more vigorous expression of Th1-type cytokines, as well as an increase in the induction of nitric oxide in the lungs of treated animals, resulting in significant reduction of bacterial numbers in the lungs of infected mice .
Metabolic Pathways
Cyclic Diguanylic Acid is involved in numerous metabolic pathways. It interacts with a multitude of enzymes and cofactors . Proteins involved in Cyclic Diguanylic Acid metabolism and regulation, such as diguanylate cyclases, phosphodiesterases, and PilZ-containing proteins, were encoded in bacterial genomes .
Transport and Distribution
Cyclic Diguanylic Acid is transported and distributed within cells and tissues . As Cyclic Diguanylic Acid is a small molecule which can diffuse easily inside the cell, there must be a mechanism for activating only specific Cyclic Diguanylic Acid-associated pathways at a given point of time while avoiding any undesirable crosstalk between the numerous Cyclic Diguanylic Acid-binding partners .
Subcellular Localization
It has been shown that the transcriptional regulator, VpsT, requires Cyclic Diguanylic Acid binding for subcellular localization and activity .
Méthodes De Préparation
Voies de synthèse et conditions réactionnelles
Le bis-(3’, 5’)-diguanosine monophosphate cyclique (disodique) peut être synthétisé à l’aide de méthodes chimiques et enzymatiques. La synthèse enzymatique est considérée comme la méthode la plus efficace. Elle implique l’utilisation de diguanylate cyclases, qui catalysent la condensation de deux molécules de guanosine-5’-triphosphate pour former du bis-(3’, 5’)-diguanosine monophosphate cyclique . Les conditions réactionnelles nécessitent généralement la présence de cations divalents tels que le magnésium ou le manganèse pour faciliter l’activité enzymatique .
Méthodes de production industrielle
Pour la production à l’échelle industrielle, la méthode enzymatique est préférée en raison de son efficacité et de sa possibilité de mise à l’échelle. Les diguanylate cyclases thermophiles, qui sont plus stables et moins sujettes à l’inhibition du produit, sont souvent utilisées. Ces enzymes peuvent produire des centaines de milligrammes de bis-(3’, 5’)-diguanosine monophosphate cyclique en utilisant de petites quantités de biocatalyseur .
Analyse Des Réactions Chimiques
Types de réactions
Le bis-(3’, 5’)-diguanosine monophosphate cyclique (disodique) subit diverses réactions chimiques, notamment l’hydrolyse et les interactions de liaison avec des récepteurs spécifiques. L’hydrolyse du bis-(3’, 5’)-diguanosine monophosphate cyclique est catalysée par les phosphodiestérases, qui le décomposent en nucléotides linéaires ou en guanosine monophosphate .
Réactifs et conditions courantes
La réaction d’hydrolyse nécessite généralement la présence de phosphodiestérases avec des domaines EAL ou HD-GYP. Ces enzymes facilitent la dégradation du bis-(3’, 5’)-diguanosine monophosphate cyclique dans des conditions physiologiques .
Principaux produits formés
Les principaux produits de la réaction d’hydrolyse sont les nucléotides linéaires tels que la 5’-phosphoguanylyl-(3’→5’)-guanosine et la guanosine monophosphate .
Applications de la recherche scientifique
Le bis-(3’, 5’)-diguanosine monophosphate cyclique (disodique) a une large gamme d’applications de recherche scientifique :
Comparaison Avec Des Composés Similaires
Le bis-(3’, 5’)-diguanosine monophosphate cyclique (disodique) est unique par rapport aux autres seconds messagers en raison de son rôle spécifique dans la signalisation bactérienne et de sa capacité à moduler les réponses immunitaires de l’hôte. Les composés similaires comprennent :
Adénosine monophosphate cyclique (AMPc) : Un autre second messager impliqué dans divers processus cellulaires chez les procaryotes et les eucaryotes.
Guanosine monophosphate cyclique (GMPc) : Une molécule de signalisation qui régule le tonus vasculaire, la croissance cellulaire et l’apoptose.
Di-adénosine monophosphate cyclique (c-di-AMP) : Un second messager impliqué dans la croissance bactérienne, le maintien de la paroi cellulaire et la virulence.
Le bis-(3’, 5’)-diguanosine monophosphate cyclique (disodique) se distingue par son double rôle dans la signalisation bactérienne et la modulation immunitaire de l’hôte, ce qui en fait une cible prometteuse pour les interventions thérapeutiques .
Propriétés
IUPAC Name |
2-amino-9-[(1S,6R,8R,9R,10S,15R,17R,18R)-17-(2-amino-6-oxo-1H-purin-9-yl)-3,9,12,18-tetrahydroxy-3,12-dioxo-2,4,7,11,13,16-hexaoxa-3λ5,12λ5-diphosphatricyclo[13.3.0.06,10]octadecan-8-yl]-1H-purin-6-one | |
---|---|---|
Source | PubChem | |
URL | https://pubchem.ncbi.nlm.nih.gov | |
Description | Data deposited in or computed by PubChem | |
InChI |
InChI=1S/C20H24N10O14P2/c21-19-25-13-7(15(33)27-19)23-3-29(13)17-9(31)11-5(41-17)1-39-45(35,36)44-12-6(2-40-46(37,38)43-11)42-18(10(12)32)30-4-24-8-14(30)26-20(22)28-16(8)34/h3-6,9-12,17-18,31-32H,1-2H2,(H,35,36)(H,37,38)(H3,21,25,27,33)(H3,22,26,28,34)/t5-,6-,9-,10-,11-,12-,17-,18-/m1/s1 | |
Source | PubChem | |
URL | https://pubchem.ncbi.nlm.nih.gov | |
Description | Data deposited in or computed by PubChem | |
InChI Key |
PKFDLKSEZWEFGL-MHARETSRSA-N | |
Source | PubChem | |
URL | https://pubchem.ncbi.nlm.nih.gov | |
Description | Data deposited in or computed by PubChem | |
Canonical SMILES |
C1C2C(C(C(O2)N3C=NC4=C3N=C(NC4=O)N)O)OP(=O)(OCC5C(C(C(O5)N6C=NC7=C6N=C(NC7=O)N)O)OP(=O)(O1)O)O | |
Source | PubChem | |
URL | https://pubchem.ncbi.nlm.nih.gov | |
Description | Data deposited in or computed by PubChem | |
Isomeric SMILES |
C1[C@@H]2[C@H]([C@H]([C@@H](O2)N3C=NC4=C3N=C(NC4=O)N)O)OP(=O)(OC[C@@H]5[C@H]([C@H]([C@@H](O5)N6C=NC7=C6N=C(NC7=O)N)O)OP(=O)(O1)O)O | |
Source | PubChem | |
URL | https://pubchem.ncbi.nlm.nih.gov | |
Description | Data deposited in or computed by PubChem | |
Molecular Formula |
C20H24N10O14P2 | |
Source | PubChem | |
URL | https://pubchem.ncbi.nlm.nih.gov | |
Description | Data deposited in or computed by PubChem | |
DSSTOX Substance ID |
DTXSID801027528 | |
Record name | Cyclic diguanylic acid | |
Source | EPA DSSTox | |
URL | https://comptox.epa.gov/dashboard/DTXSID801027528 | |
Description | DSSTox provides a high quality public chemistry resource for supporting improved predictive toxicology. | |
Molecular Weight |
690.4 g/mol | |
Source | PubChem | |
URL | https://pubchem.ncbi.nlm.nih.gov | |
Description | Data deposited in or computed by PubChem | |
CAS No. |
61093-23-0 | |
Record name | c-Di-GMP | |
Source | CAS Common Chemistry | |
URL | https://commonchemistry.cas.org/detail?cas_rn=61093-23-0 | |
Description | CAS Common Chemistry is an open community resource for accessing chemical information. Nearly 500,000 chemical substances from CAS REGISTRY cover areas of community interest, including common and frequently regulated chemicals, and those relevant to high school and undergraduate chemistry classes. This chemical information, curated by our expert scientists, is provided in alignment with our mission as a division of the American Chemical Society. | |
Explanation | The data from CAS Common Chemistry is provided under a CC-BY-NC 4.0 license, unless otherwise stated. | |
Retrosynthesis Analysis
AI-Powered Synthesis Planning: Our tool employs the Template_relevance Pistachio, Template_relevance Bkms_metabolic, Template_relevance Pistachio_ringbreaker, Template_relevance Reaxys, Template_relevance Reaxys_biocatalysis model, leveraging a vast database of chemical reactions to predict feasible synthetic routes.
One-Step Synthesis Focus: Specifically designed for one-step synthesis, it provides concise and direct routes for your target compounds, streamlining the synthesis process.
Accurate Predictions: Utilizing the extensive PISTACHIO, BKMS_METABOLIC, PISTACHIO_RINGBREAKER, REAXYS, REAXYS_BIOCATALYSIS database, our tool offers high-accuracy predictions, reflecting the latest in chemical research and data.
Strategy Settings
Precursor scoring | Relevance Heuristic |
---|---|
Min. plausibility | 0.01 |
Model | Template_relevance |
Template Set | Pistachio/Bkms_metabolic/Pistachio_ringbreaker/Reaxys/Reaxys_biocatalysis |
Top-N result to add to graph | 6 |
Feasible Synthetic Routes
Q1: What is cyclic diguanylic acid (c-di-GMP) and what is its significance in bacteria?
A: Cyclic diguanylic acid (this compound) is a ubiquitous second messenger molecule found in bacteria. [] It plays a crucial role in regulating a wide range of cellular processes in bacteria, primarily acting as a molecular switch between motile (free-swimming) and sessile (surface-attached) lifestyles. []
Q2: How is this compound synthesized and degraded within bacterial cells?
A: this compound is synthesized from two molecules of GTP by enzymes called diguanylate cyclases (DGCs), which contain a characteristic GGDEF domain. [, ] Conversely, this compound is degraded into linear pGpG or two GMP molecules by enzymes called phosphodiesterases (PDEs), which possess either EAL or HD-GYP domains. [, ]
Q3: How does the number of genes encoding this compound-modulating enzymes affect bacterial adaptation?
A: Bacteria exhibit significant variation in the number of genes encoding GGDEF, EAL, and HD-GYP proteins. [] A greater number of these genes translate into a more complex this compound signaling network, enabling bacteria to fine-tune the regulation of target genes and optimize their adaptation to diverse environments. [, ]
Q4: What is the general mechanism of action of this compound in bacterial signaling pathways?
A: this compound exerts its regulatory effects by binding to various effector proteins, such as transcription factors, enzymes, and multimeric protein complexes. [] This binding event triggers downstream signaling cascades that ultimately control a wide range of bacterial behaviors, including biofilm formation, motility, virulence, and cell cycle progression.
Q5: How does this compound influence biofilm formation?
A: High intracellular levels of this compound generally promote biofilm formation. [, , , ] This is achieved through multiple mechanisms, including:
- Repression of motility: this compound inhibits bacterial motility by reducing flagellar synthesis and function, thereby promoting surface attachment. [, , , ] For instance, in Pseudomonas aeruginosa, elevated this compound levels lead to reduced flagellar motility and enhanced biofilm formation. []
- Induction of biofilm matrix production: this compound stimulates the production of extracellular polysaccharide (EPS), a major component of the biofilm matrix. [, , ] For example, in Pseudomonas aeruginosa, the diguanylate cyclase RoeA promotes EPS production and contributes to biofilm formation. []
- Regulation of adhesin expression: this compound can modulate the expression of bacterial adhesins, which mediate attachment to surfaces and other cells. [] In Pseudomonas putida, this compound/FleQ regulates the transcription of lapE, which encodes an outer membrane pore protein involved in adhesin secretion and biofilm formation. []
Q6: How does this compound impact bacterial motility?
A: Elevated this compound levels typically suppress bacterial motility, primarily by inhibiting flagellar synthesis and function. [, , , ] For example, in Myxococcus xanthus, this compound regulates type IV pilus-dependent motility. [] Conversely, low this compound levels generally promote motility by facilitating flagellar biosynthesis and rotation. []
Q7: Does this compound regulate virulence in pathogenic bacteria?
A: The role of this compound in bacterial virulence is complex and can be context-dependent. While generally considered an inhibitor of virulence factors, exceptions to this paradigm exist. []
- Virulence suppression: In some pathogens, high this compound levels correlate with reduced virulence, often indirectly through the inhibition of motility and biofilm dispersal. [, , ] In Vibrio cholerae, for example, this compound directly inhibits the expression of cholera toxin. []
- Virulence enhancement: Conversely, in certain pathogens, this compound contributes to virulence by promoting host colonization, persistence, and immune evasion. [, , ] For instance, in Ehrlichia chaffeensis, this compound signaling is essential for bacterial invasion of human monocytes. []
Q8: Can you provide examples of specific this compound effector proteins and their functions?
A8: Numerous this compound effector proteins have been identified, each contributing to specific downstream effects:
- FleQ: In Pseudomonas species, FleQ acts as a transcriptional regulator, controlling the expression of biofilm- and flagellum-related genes in response to this compound. []
- BldD: In Streptomyces, BldD dimerizes upon binding to this compound, impacting the transcription of genes involved in differentiation and development. []
- PilZ domain proteins: These proteins, often containing one or more PilZ domains, are common this compound receptors involved in various cellular processes. [, , ] For instance, in Myxococcus xanthus, PlpA, PixA, and PixB are PilZ domain proteins with distinct roles in regulating motility and development. []
- MshE: This ATPase, associated with type IV pilus, binds this compound via its N-terminal T2SSE_N domain, influencing pilus-dependent processes. []
Q9: How is this compound signaling specificity achieved in bacteria with complex this compound networks?
A9: Maintaining signaling specificity in bacteria with numerous DGCs and PDEs is crucial for proper cellular function. Several mechanisms contribute to this specificity:
- Spatiotemporal regulation: The localization and timing of this compound synthesis and degradation are tightly controlled. [, , , ] Specific DGCs and PDEs are often localized to distinct cellular compartments or expressed under specific conditions, ensuring that this compound signals are directed to the appropriate downstream effectors.
- Protein-protein interactions: Direct interactions between this compound-metabolizing enzymes and their cognate effectors contribute to signaling fidelity. [, , , ] These interactions can enhance the local concentration of this compound near its target, preventing cross-talk between different pathways.
- Feedback regulation: this compound can modulate the activity of DGCs and PDEs through feedback mechanisms, fine-tuning its own intracellular levels. [] This feedback control helps to maintain this compound homeostasis and prevent aberrant signaling.
Q10: How does the study of this compound signaling contribute to our understanding of bacterial pathogenesis and potential therapeutic interventions?
A10: Understanding the intricacies of this compound signaling in pathogenic bacteria holds significant promise for developing novel therapeutic strategies:
- Anti-biofilm agents: Disrupting this compound signaling pathways essential for biofilm formation could lead to new treatments for chronic and recurrent infections. [, ] Targeting DGCs, PDEs, or this compound effector proteins could potentially prevent biofilm formation or disperse existing biofilms, rendering bacteria more susceptible to antibiotics and host immune responses.
- Antivirulence therapies: Modulating this compound signaling in pathogens where it promotes virulence could attenuate their pathogenicity and enhance the efficacy of existing treatments. [] Inhibiting specific DGCs or activating specific PDEs involved in virulence regulation could potentially disarm pathogens without directly killing them, thereby minimizing the emergence of antibiotic resistance.
- Probiotic interventions: Manipulating this compound signaling in beneficial bacteria could enhance their ability to colonize the host and outcompete pathogens. [] Increasing this compound levels in probiotics could potentially enhance their biofilm-forming capacity, improving their persistence and effectiveness in the gut.
Avertissement et informations sur les produits de recherche in vitro
Veuillez noter que tous les articles et informations sur les produits présentés sur BenchChem sont destinés uniquement à des fins informatives. Les produits disponibles à l'achat sur BenchChem sont spécifiquement conçus pour des études in vitro, qui sont réalisées en dehors des organismes vivants. Les études in vitro, dérivées du terme latin "in verre", impliquent des expériences réalisées dans des environnements de laboratoire contrôlés à l'aide de cellules ou de tissus. Il est important de noter que ces produits ne sont pas classés comme médicaments et n'ont pas reçu l'approbation de la FDA pour la prévention, le traitement ou la guérison de toute condition médicale, affection ou maladie. Nous devons souligner que toute forme d'introduction corporelle de ces produits chez les humains ou les animaux est strictement interdite par la loi. Il est essentiel de respecter ces directives pour assurer la conformité aux normes légales et éthiques en matière de recherche et d'expérimentation.