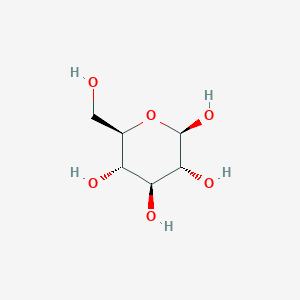
β-D-glucose
Vue d'ensemble
Description
Beta-D-Glucose is a form of glucose, a simple sugar that serves as a primary source of energy for living organisms . It is naturally occurring and is found in fruits and other parts of plants in its free state . Beta-D-Glucose is a D-Glucopyranose with beta configuration at the anomeric centre . It has a role as an epitope and a mouse metabolite .
Synthesis Analysis
The synthesis of beta-D-Glucose is connected to the way of extraction from the natural source . Various studies have reported the relationship between the molecular structure of beta-D-Glucose and its functionality . The structural characteristics of beta-D-Glucose, including specific glycosidic linkages, monosaccharide compositions, molecular weight, and chain conformation, were reported to affect its physiochemical and biological properties .Molecular Structure Analysis
Beta-D-Glucose contains total 24 bond(s); 12 non-H bond(s), 1 rotatable bond(s), 1 six-membered ring(s), 5 hydroxyl group(s), 1 primary alcohol(s), 3 secondary alcohol(s), and 1 ether(s) (aliphatic) . The two-dimensional graphical representation of these isomeric glucose structures shows that the beta-D-Glucose has (1-hydroxyl) and (4-hydroxyl) orientations on the same side .Chemical Reactions Analysis
Beta-D-Glucose has been reported to have various chemical reactions. For instance, the products released from the digestion of purified beta-D-Glucose are mainly cellobiosyl- (1→3)-beta-D-Glucose and cellotriosyl- (1→3)-beta-D-Glucose, with smaller amounts of cellotetraosyl- (1→3)-beta-D-Glucose and cellopentaosyl- (1→3)-beta-D-Glucose .Physical and Chemical Properties Analysis
The high molecular weight (Mw) and high viscosity of beta-D-Glucose are responsible for its hypocholesterolemic and hypoglycemic properties . The inherent gel-forming property and high viscosity of beta-D-Glucose lead to the production of low-fat foods with improved textural properties .Applications De Recherche Scientifique
Production de biocarburants
Le β-D-glucose est crucial dans la saccharification de la biomasse lignocellulosique, une étape clé dans la production de bioéthanol, une source d'énergie renouvelable. Des enzymes comme les β-glucosidases (BGL) décomposent la cellulose en glucose, qui peut ensuite être fermenté en éthanol .
Industrie alimentaire et des boissons
Dans l'industrie alimentaire, les BGL améliorent les saveurs dans la production de vin et de jus en décomposant les glucosides en composés aromatiques. Elles sont également utilisées pour améliorer la digestion de la cellulose dans les aliments pour animaux, contribuant à une meilleure absorption des nutriments .
Applications pharmaceutiques
Les dérivés du this compound sont utilisés dans les produits pharmaceutiques pour leur activité enzymatique, qui peut aider dans les systèmes d'administration de médicaments et la synthèse de molécules complexes .
Processus de détoxification
Les BGL jouent un rôle dans la détoxification des glucosides cyanogènes dans les cultures alimentaires, les rendant plus sûres pour la consommation et réduisant le risque d'empoisonnement au cyanure .
Recyclage du papier usagé
L'activité enzymatique des BGL est utilisée dans le recyclage du papier usagé pour décomposer les fibres de cellulose, aidant au processus de repulpage du papier et contribuant à des pratiques de gestion durable des déchets .
Industrie textile
Dans les textiles, les cellulases, y compris les β-glucosidases, sont utilisées pour modifier les propriétés de surface des tissus, améliorant la douceur et créant des effets désirés comme des aspects délavés à la pierre sans utiliser de produits chimiques agressifs .
Industrie des détergents
Les enzymes this compound sont ajoutées aux détergents à lessive pour aider à décomposer les taches et les saletés sur les tissus, améliorant l'efficacité du nettoyage et réduisant l'impact environnemental en permettant le lavage à des températures plus basses .
Recherche biochimique
Le this compound est utilisé comme substrat dans les essais biochimiques pour étudier la cinétique enzymatique et les voies métaboliques, fournissant des informations sur les processus cellulaires et les mécanismes des maladies .
Mécanisme D'action
Target of Action
Beta-D-Glucose, a naturally occurring compound found in fruits and other parts of plants , interacts with various targets in living organisms. It is known to interact with several enzymes such as DNA, Chondroitinase-B, Endoglucanase 5A, Lactase-like protein, Glycogen phosphorylase, Xylose isomerase, Glucokinase, Ferrichrome-iron receptor, and Hexokinase-1 . These enzymes play crucial roles in various biochemical processes, including energy production, metabolism, and cellular function .
Mode of Action
Beta-D-Glucose’s mode of action is primarily through its interaction with these enzymes. For instance, Glucokinase, an enzyme found in the liver and beta cells of the pancreas, catalyzes the initial step in the utilization of glucose at physiological glucose concentration . Beta-D-Glucose is also known to interact with Hexokinase-1, another key enzyme involved in the glycolytic pathway . The interaction of Beta-D-Glucose with these enzymes leads to various biochemical changes within the cell .
Biochemical Pathways
Beta-D-Glucose plays a significant role in the glycolytic pathway, also known as the Meyerhoff, Embden, Parnas pathway . This pathway involves the anaerobic conversion of glucose to pyruvate, providing the cell with ATP under anaerobic conditions and supplying precursors for the biosynthesis of proteins, lipids, nucleic acids, and polysaccharides . The enzymes involved in glycolysis, including Hexokinase and Phosphofructokinase 1 (PFK 1), are regulated by small molecules, and Beta-D-Glucose plays a crucial role in this regulation .
Pharmacokinetics
While specific pharmacokinetic data on Beta-D-Glucose is limited, it is known that the compound exhibits dose-proportional pharmacokinetics without apparent accumulation in the body . This suggests that Beta-D-Glucose is metabolized and excreted efficiently, impacting its bioavailability.
Result of Action
The primary result of Beta-D-Glucose’s action is the provision of energy for living organisms . By participating in glycolysis, Beta-D-Glucose contributes to the production of ATP, the primary energy currency of the cell . Additionally, the interaction of Beta-D-Glucose with various enzymes can lead to changes in cellular function and metabolism .
Action Environment
The action of Beta-D-Glucose is influenced by various environmental factors. For instance, the activity of Beta-D-Glucose-degrading enzymes can be affected by factors such as temperature and pH . Additionally, the presence of other molecules can impact the action of Beta-D-Glucose. For example, in the presence of certain microbial structures, plant Beta-glucanases can hydrolyze Beta-glucans, triggering the activation of global responses .
Analyse Biochimique
Biochemical Properties
Beta-D-Glucose plays a crucial role in various biochemical reactions. It is the primary source of energy for cells and is metabolized through glycolysis. Beta-D-Glucose interacts with various enzymes, such as hexokinase and glucokinase, which phosphorylate glucose to glucose-6-phosphate, the first step in most glucose metabolism pathways .
Cellular Effects
Beta-D-Glucose impacts various types of cells and cellular processes. It influences cell function by providing the energy needed for cellular processes. It also impacts cell signaling pathways, gene expression, and cellular metabolism. For instance, high levels of Beta-D-Glucose can lead to increased insulin production in beta cells of the pancreas .
Molecular Mechanism
The molecular mechanism of Beta-D-Glucose involves its conversion to glucose-6-phosphate by the action of hexokinase or glucokinase. This is a crucial step as it keeps glucose inside the cell and makes it available for various metabolic pathways .
Temporal Effects in Laboratory Settings
In laboratory settings, Beta-D-Glucose shows stability over time. Its effects on cellular function can vary depending on the concentration and duration of exposure .
Dosage Effects in Animal Models
In animal models, the effects of Beta-D-Glucose can vary with different dosages. High doses of glucose can lead to hyperglycemia and insulin resistance, while low doses may not provide sufficient energy for normal cellular function .
Metabolic Pathways
Beta-D-Glucose is involved in several metabolic pathways, including glycolysis, gluconeogenesis, and the pentose phosphate pathway. It interacts with various enzymes in these pathways, such as hexokinase, phosphofructokinase, and glucose-6-phosphate dehydrogenase .
Transport and Distribution
Beta-D-Glucose is transported into cells via glucose transporters (GLUTs). Once inside the cell, it is phosphorylated to glucose-6-phosphate to prevent it from leaving the cell .
Subcellular Localization
Inside the cell, Beta-D-Glucose is primarily found in the cytoplasm where glycolysis takes place. It can also be found in the mitochondria where it can be further metabolized to produce ATP .
Propriétés
IUPAC Name |
6-(hydroxymethyl)oxane-2,3,4,5-tetrol | |
---|---|---|
Details | Computed by Lexichem TK 2.7.0 (PubChem release 2021.05.07) | |
Source | PubChem | |
URL | https://pubchem.ncbi.nlm.nih.gov | |
Description | Data deposited in or computed by PubChem | |
InChI |
InChI=1S/C6H12O6/c7-1-2-3(8)4(9)5(10)6(11)12-2/h2-11H,1H2 | |
Details | Computed by InChI 1.0.6 (PubChem release 2021.05.07) | |
Source | PubChem | |
URL | https://pubchem.ncbi.nlm.nih.gov | |
Description | Data deposited in or computed by PubChem | |
InChI Key |
WQZGKKKJIJFFOK-UHFFFAOYSA-N | |
Details | Computed by InChI 1.0.6 (PubChem release 2021.05.07) | |
Source | PubChem | |
URL | https://pubchem.ncbi.nlm.nih.gov | |
Description | Data deposited in or computed by PubChem | |
Canonical SMILES |
C(C1C(C(C(C(O1)O)O)O)O)O | |
Details | Computed by OEChem 2.3.0 (PubChem release 2021.05.07) | |
Source | PubChem | |
URL | https://pubchem.ncbi.nlm.nih.gov | |
Description | Data deposited in or computed by PubChem | |
Molecular Formula |
C6H12O6 | |
Details | Computed by PubChem 2.1 (PubChem release 2021.05.07) | |
Source | PubChem | |
URL | https://pubchem.ncbi.nlm.nih.gov | |
Description | Data deposited in or computed by PubChem | |
DSSTOX Substance ID |
DTXSID80858960 | |
Record name | Hexopyranose | |
Source | EPA DSSTox | |
URL | https://comptox.epa.gov/dashboard/DTXSID80858960 | |
Description | DSSTox provides a high quality public chemistry resource for supporting improved predictive toxicology. | |
Molecular Weight |
180.16 g/mol | |
Details | Computed by PubChem 2.1 (PubChem release 2021.05.07) | |
Source | PubChem | |
URL | https://pubchem.ncbi.nlm.nih.gov | |
Description | Data deposited in or computed by PubChem | |
CAS No. |
42752-07-8, 579-36-2, 2280-44-6, 39392-65-9, 10257-28-0 | |
Record name | Hexopyranose | |
Source | CAS Common Chemistry | |
URL | https://commonchemistry.cas.org/detail?cas_rn=42752-07-8 | |
Description | CAS Common Chemistry is an open community resource for accessing chemical information. Nearly 500,000 chemical substances from CAS REGISTRY cover areas of community interest, including common and frequently regulated chemicals, and those relevant to high school and undergraduate chemistry classes. This chemical information, curated by our expert scientists, is provided in alignment with our mission as a division of the American Chemical Society. | |
Explanation | The data from CAS Common Chemistry is provided under a CC-BY-NC 4.0 license, unless otherwise stated. | |
Record name | D-Allopyranose | |
Source | DTP/NCI | |
URL | https://dtp.cancer.gov/dtpstandard/servlet/dwindex?searchtype=NSC&outputformat=html&searchlist=144657 | |
Description | The NCI Development Therapeutics Program (DTP) provides services and resources to the academic and private-sector research communities worldwide to facilitate the discovery and development of new cancer therapeutic agents. | |
Explanation | Unless otherwise indicated, all text within NCI products is free of copyright and may be reused without our permission. Credit the National Cancer Institute as the source. | |
Record name | NSC287045 | |
Source | DTP/NCI | |
URL | https://dtp.cancer.gov/dtpstandard/servlet/dwindex?searchtype=NSC&outputformat=html&searchlist=287045 | |
Description | The NCI Development Therapeutics Program (DTP) provides services and resources to the academic and private-sector research communities worldwide to facilitate the discovery and development of new cancer therapeutic agents. | |
Explanation | Unless otherwise indicated, all text within NCI products is free of copyright and may be reused without our permission. Credit the National Cancer Institute as the source. | |
Record name | NSC274237 | |
Source | DTP/NCI | |
URL | https://dtp.cancer.gov/dtpstandard/servlet/dwindex?searchtype=NSC&outputformat=html&searchlist=274237 | |
Description | The NCI Development Therapeutics Program (DTP) provides services and resources to the academic and private-sector research communities worldwide to facilitate the discovery and development of new cancer therapeutic agents. | |
Explanation | Unless otherwise indicated, all text within NCI products is free of copyright and may be reused without our permission. Credit the National Cancer Institute as the source. | |
Record name | D-galactose | |
Source | DTP/NCI | |
URL | https://dtp.cancer.gov/dtpstandard/servlet/dwindex?searchtype=NSC&outputformat=html&searchlist=8102 | |
Description | The NCI Development Therapeutics Program (DTP) provides services and resources to the academic and private-sector research communities worldwide to facilitate the discovery and development of new cancer therapeutic agents. | |
Explanation | Unless otherwise indicated, all text within NCI products is free of copyright and may be reused without our permission. Credit the National Cancer Institute as the source. | |
Record name | Hexopyranose | |
Source | EPA DSSTox | |
URL | https://comptox.epa.gov/dashboard/DTXSID80858960 | |
Description | DSSTox provides a high quality public chemistry resource for supporting improved predictive toxicology. | |
Synthesis routes and methods I
Procedure details
Synthesis routes and methods II
Procedure details
Retrosynthesis Analysis
AI-Powered Synthesis Planning: Our tool employs the Template_relevance Pistachio, Template_relevance Bkms_metabolic, Template_relevance Pistachio_ringbreaker, Template_relevance Reaxys, Template_relevance Reaxys_biocatalysis model, leveraging a vast database of chemical reactions to predict feasible synthetic routes.
One-Step Synthesis Focus: Specifically designed for one-step synthesis, it provides concise and direct routes for your target compounds, streamlining the synthesis process.
Accurate Predictions: Utilizing the extensive PISTACHIO, BKMS_METABOLIC, PISTACHIO_RINGBREAKER, REAXYS, REAXYS_BIOCATALYSIS database, our tool offers high-accuracy predictions, reflecting the latest in chemical research and data.
Strategy Settings
Precursor scoring | Relevance Heuristic |
---|---|
Min. plausibility | 0.01 |
Model | Template_relevance |
Template Set | Pistachio/Bkms_metabolic/Pistachio_ringbreaker/Reaxys/Reaxys_biocatalysis |
Top-N result to add to graph | 6 |
Feasible Synthetic Routes
Q1: What is the molecular formula and weight of β-D-glucose?
A1: this compound shares the same molecular formula as its isomer α-D-glucose: C6H12O6. Its molecular weight is 180.16 g/mol.
Q2: How does the structure of this compound differ from α-D-glucose?
A2: Both are anomers, differing in the orientation of the hydroxyl group (-OH) at the anomeric carbon (C1). In this compound, the -OH group on C1 is oriented in the equatorial position, while in α-D-glucose, it is in the axial position. This seemingly small difference leads to distinct chemical properties and biological activities.
Q3: Are there spectroscopic techniques to differentiate between the anomers of D-glucose?
A3: Yes, techniques like Nuclear Magnetic Resonance (NMR) spectroscopy can distinguish between α and β anomers. For instance, the anomeric proton (H1) in this compound typically exhibits a characteristic chemical shift in the 1H NMR spectrum. []
Q4: How does this compound interact with enzymes involved in glucose metabolism?
A4: this compound exhibits distinct interactions with various enzymes involved in glucose metabolism. For instance, studies on hexokinase, an enzyme catalyzing the first step of glycolysis, demonstrate anomeric preference. Bovine heart hexokinase displays a higher maximal velocity with this compound, while yeast hexokinase shows a higher affinity for α-D-glucose. Interestingly, these anomeric preferences are temperature-dependent. [] []
Q5: Do these anomeric preferences translate to differences in metabolic fate?
A5: Yes, studies in rat adipocytes revealed that while α-D-glucose utilization was higher overall, the fraction of glucose catabolized via the pentose phosphate pathway was higher with this compound. These findings highlight the anomeric specificity of glucose metabolism even when exposed to equilibrated D-glucose. []
Q6: Are there differences in how this compound is transported compared to α-D-glucose?
A6: Research on human red blood cells suggests that both anomers are transported by the hexose transport system without any significant difference. This was observed at both low (0.6 degrees C) and physiological (36.6 degrees C) temperatures, suggesting no anomeric preference for the transporter. []
Q7: Does this compound play a role in the synthesis of specialized metabolites?
A7: Yes, this compound serves as a precursor for the synthesis of various compounds. In Lycopersicon pennellii, a wild tomato species, this compound is involved in the biosynthesis of 2,3,4-tri-O-acylglucoses. These compounds, secreted by glandular trichomes, possess short to medium-chain fatty acids and contribute to plant defense mechanisms. The biosynthesis involves the activation of fatty acids to form 1-O-acyl-β-D-glucopyranose derivatives, which then undergo transacylation reactions to generate the final tri-O-acylglucoses. [] []
Q8: Can this compound act as a stabilizing agent in nanoparticle synthesis?
A8: Absolutely. Research demonstrates the use of this compound as both a reducing and capping agent in the "green" synthesis of gold nanoparticles. These nanoparticles, averaging 8.2 nm in diameter, were successfully extracted into an organic phase and used to create thin films and ordered arrays. [] []
Q9: Has this compound been utilized in the development of catalytic systems?
A9: Yes, this compound-stabilized gold nanoparticles have demonstrated catalytic activity. They effectively catalyze the reduction of 4-nitrophenol in the presence of NaBH4, a reaction that is otherwise unfeasible with NaBH4 alone. This highlights the potential of these bio-synthesized nanoparticles for catalytic applications. []
Q10: What is the significance of the this compound scaffold in drug discovery?
A10: The this compound scaffold holds promise as a β-turn mimetic in drug design. Research has shown that this scaffold can mimic the β-turn in somatostatin (SRIF) and its synthetic analog L-363,301, both of which bind to SRIF receptors. This discovery paved the way for the development of non-peptidic this compound-based analogs with agonist activity at SRIF receptors. []
Avertissement et informations sur les produits de recherche in vitro
Veuillez noter que tous les articles et informations sur les produits présentés sur BenchChem sont destinés uniquement à des fins informatives. Les produits disponibles à l'achat sur BenchChem sont spécifiquement conçus pour des études in vitro, qui sont réalisées en dehors des organismes vivants. Les études in vitro, dérivées du terme latin "in verre", impliquent des expériences réalisées dans des environnements de laboratoire contrôlés à l'aide de cellules ou de tissus. Il est important de noter que ces produits ne sont pas classés comme médicaments et n'ont pas reçu l'approbation de la FDA pour la prévention, le traitement ou la guérison de toute condition médicale, affection ou maladie. Nous devons souligner que toute forme d'introduction corporelle de ces produits chez les humains ou les animaux est strictement interdite par la loi. Il est essentiel de respecter ces directives pour assurer la conformité aux normes légales et éthiques en matière de recherche et d'expérimentation.