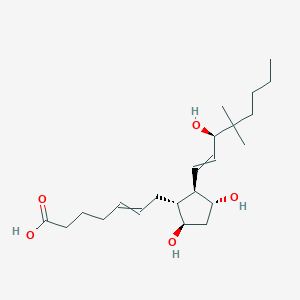
16,16-diméthyl-PGF2β
Vue d'ensemble
Description
16,16-Dimethyl-PGF2beta is a synthetic analog of prostaglandin F2beta, a type of prostanoid. Prostaglandins are lipid compounds that have diverse hormone-like effects in animals. 16,16-Dimethyl-PGF2beta mimics the activity of natural prostaglandins and has been extensively studied for its biological activity and potential therapeutic applications.
Applications De Recherche Scientifique
Hematopoietic Stem Cell Amplification
One of the most notable applications of dmPGE2 is its ability to amplify hematopoietic stem cells (HSCs). Research has demonstrated that dmPGE2 can significantly increase HSC numbers and enhance their functionality. In a study involving zebrafish embryos, dmPGE2 treatment resulted in a remarkable 300-400% increase in blood stem cells . This discovery has implications for bone marrow transplantation, particularly for patients with blood cancers such as leukemia and lymphoma.
Case Study: Clinical Trials
A Phase Ib clinical trial assessed the effects of dmPGE2 on umbilical cord blood transplantation. Twelve patients received both treated and untreated cord blood units. Results indicated that all patients had immune system reconstitution, with 10 out of 12 showing blood formation exclusively from the dmPGE2-treated unit . The ongoing Phase II trial aims to further evaluate the efficacy of this treatment.
Radioprotection
dmPGE2 has been shown to protect against radiation-induced damage, particularly in hematopoietic tissues. Studies indicate that pre-treatment with dmPGE2 enhances the survival of clonogenic cells in irradiated mice. For instance, the lethal dose for 50% survival (LD50) increased significantly when mice were treated with dmPGE2 prior to radiation exposure . This suggests potential applications in mitigating radiation injuries during cancer treatments or nuclear incidents.
Table 1: Radioprotection Efficacy of dmPGE2
Treatment | LD50 (Gy) | Survival Rate (%) |
---|---|---|
Control | 16.3 | 50 |
dmPGE2 | 20.25 | Increased |
Gastrointestinal Protection
The protective effects of dmPGE2 extend to gastrointestinal health, where it has been found to safeguard the intestinal lining from various stressors, including radiation and non-steroidal anti-inflammatory drugs (NSAIDs). In murine models, dmPGE2 administration prior to radiation exposure improved intestinal stem cell survival . This application is particularly relevant for patients undergoing chemotherapy who are at risk of gastrointestinal toxicity.
Pharmacokinetics and Biodistribution
Understanding the pharmacokinetics (PK) and biodistribution of dmPGE2 is crucial for its effective application in clinical settings. A study evaluated the PK profile of dmPGE2 in both irradiated and non-irradiated mice, revealing significant differences in absorption and metabolism across species . Such insights are vital for translating animal model findings to human clinical applications.
Table 2: Pharmacokinetic Parameters of dmPGE2
Parameter | C57BL/6J Mice | JDO Mice |
---|---|---|
AUC0–Inf (ng·h/ml) | 112.50 | 114.48 |
Cmax (ng/ml) | 44.53 | 63.96 |
t1/2 (h) | 1.80 | 1.10 |
Mécanisme D'action
Target of Action
16,16-Dimethyl prostaglandin F2beta (16,16-dimethyl-PGF2beta) is a synthetic analog of prostaglandin F2beta . It primarily targets prostanoid receptors , which are a group of G-protein coupled receptors . These receptors play a crucial role in mediating the physiological effects of prostaglandins and related compounds .
Mode of Action
16,16-dimethyl-PGF2beta interacts with its prostanoid receptors, triggering a cascade of intracellular events . The exact nature of these interactions and the resulting changes are complex and can vary depending on the specific receptor subtype and the cellular context .
Biochemical Pathways
The interaction of 16,16-dimethyl-PGF2beta with its receptors affects various biochemical pathways. These pathways are involved in a wide range of physiological processes, including inflammation, pain perception, and the regulation of blood pressure . The downstream effects of these pathways can have significant impacts on human health and disease .
Pharmacokinetics
It is known to be a metabolically stable analog of pgf2beta , suggesting that it may have improved bioavailability compared to the natural compound .
Result of Action
The molecular and cellular effects of 16,16-dimethyl-PGF2beta’s action are diverse and depend on the specific context. For example, it has been shown to prevent bronchospasm in asthmatics . Additionally, it has been found to mediate chromatin flexibility at hematopoietic stem and progenitor cell (HSPC)-specific enhancers through histone-variant H2A.Z acetylation .
Action Environment
The action, efficacy, and stability of 16,16-dimethyl-PGF2beta can be influenced by various environmental factors. These can include the presence of other signaling molecules, the specific cellular and tissue context, and potentially even factors such as diet and lifestyle . .
Analyse Biochimique
Biochemical Properties
16,16-Dimethyl Prostaglandin F2beta is a prostanoid . Prostanoids are a subclass of eicosanoids produced by oxidation of 20-carbon essential fatty acids (EFAs) that are commonly incorporated within membrane phospholipids .
Cellular Effects
16,16-Dimethyl Prostaglandin F2beta exerts diverse effects on cell proliferation, apoptosis, angiogenesis, inflammation, and immune surveillance . These effects are most prominent in gastrointestinal cancers, likely due to the constant exposure to dietary and environmental insults and the intrinsic role of prostaglandins in tissue homeostasis .
Molecular Mechanism
The molecular mechanism of 16,16-Dimethyl Prostaglandin F2beta is not fully understood. It is known to mimic the activity of natural prostaglandins, which are involved in a wide range of biological effects associated with inflammation and cancer .
Dosage Effects in Animal Models
The effects of 16,16-Dimethyl Prostaglandin F2beta vary with different dosages in animal models . It has been observed that stable, synthetic analogues of prostaglandins, such as 16,16-Dimethyl Prostaglandin F2beta, after oral intake, give a dose-dependent and sustained inhibition of the basal and stimulated gastric acid secretion .
Metabolic Pathways
16,16-Dimethyl Prostaglandin F2beta is involved in the metabolic pathways of prostaglandins . Prostaglandins are synthesized by the sequential actions of a panel of highly specific enzymes .
Méthodes De Préparation
Synthetic Routes and Reaction Conditions
The synthesis of 16,16-Dimethyl-PGF2beta involves multiple steps, starting from readily available starting materials. The key steps include the formation of the cyclopentane ring, introduction of hydroxyl groups, and the addition of the dimethyl groups at the 16th position. The reaction conditions typically involve the use of strong acids or bases, protective groups to prevent unwanted reactions, and various purification techniques to isolate the desired product .
Industrial Production Methods
Industrial production of 16,16-Dimethyl-PGF2beta follows similar synthetic routes but on a larger scale. The process is optimized for efficiency and yield, often involving automated systems for precise control of reaction conditions. The use of high-purity reagents and advanced purification methods ensures the production of pharmaceutical-grade compounds .
Analyse Des Réactions Chimiques
Types of Reactions
16,16-Dimethyl-PGF2beta undergoes various chemical reactions, including:
Oxidation: The hydroxyl groups can be oxidized to form ketones or aldehydes.
Reduction: The carbonyl groups can be reduced to hydroxyl groups.
Substitution: The hydroxyl groups can be substituted with other functional groups.
Common Reagents and Conditions
Oxidation: Common oxidizing agents include potassium permanganate and chromium trioxide.
Reduction: Reducing agents such as lithium aluminum hydride and sodium borohydride are used.
Substitution: Reagents like thionyl chloride and phosphorus tribromide are used for substitution reactions.
Major Products Formed
The major products formed from these reactions depend on the specific conditions and reagents used. For example, oxidation of the hydroxyl groups can lead to the formation of ketones, while reduction of the carbonyl groups can yield alcohols .
Comparaison Avec Des Composés Similaires
16,16-Dimethyl-PGF2beta is unique among prostaglandin analogs due to its enhanced stability and prolonged biological activity. Similar compounds include:
Prostaglandin F2alpha: A natural prostaglandin with similar biological activity but less stability.
16,16-Dimethyl-PGE2: Another synthetic analog with different receptor selectivity and therapeutic applications.
Carboprost: A synthetic analog used for similar medical applications but with different pharmacokinetic properties.
Activité Biologique
16,16-Dimethyl-PGF2beta (also known as 16,16-dimethyl prostaglandin F2beta) is a synthetic analog of prostaglandin F2alpha (PGF2α), which is a naturally occurring compound involved in various physiological processes. This article explores the biological activity of 16,16-dimethyl-PGF2beta, focusing on its mechanisms of action, effects on different biological systems, and potential therapeutic applications.
Receptor Interaction:
16,16-Dimethyl-PGF2beta primarily exerts its biological effects by interacting with specific receptors in the body. These include:
- Prostaglandin F2α Receptors (FP): Activation of these receptors leads to various cellular responses, including smooth muscle contraction and modulation of inflammatory processes .
- EP Receptors: It may also interact with prostaglandin E receptors, influencing hematopoietic processes and stem cell differentiation .
Biochemical Pathways:
The compound is known to alter several biochemical pathways:
- Inhibition of Apoptosis: Studies indicate that 16,16-dimethyl-PGF2beta can protect cells from apoptosis induced by various stressors, including radiation .
- Stimulation of Cell Proliferation: This compound has been shown to enhance the survival and proliferation of intestinal stem cells following radiation exposure, suggesting a protective role in gastrointestinal mucosa .
Radioprotection
Research indicates that 16,16-dimethyl-PGF2beta significantly enhances the survival of mouse intestinal stem cells exposed to radiation. Key findings include:
- Increased Clonogenic Survival: Mice treated with this compound before irradiation exhibited an increase in the shoulder of the intestinal clonogenic cell survival curve. The D0 value (the dose required to reduce cell survival to 37%) increased from 1.10 Gy in controls to 1.58 Gy in treated groups .
- Hematopoietic Protection: The compound improved hematopoietic colony-forming unit survival, suggesting its potential use in protecting against radiation-induced damage in bone marrow .
Effects on Embryonic Stem Cells
Studies have demonstrated that 16,16-dimethyl-PGF2beta can enhance hematopoietic colony formation from embryonic stem cells (ESCs). This suggests its role in promoting differentiation and proliferation in stem cell populations, which could have implications for regenerative medicine and transplantation therapies .
Comparative Analysis with Prostaglandin F2alpha
A comparison between 16,16-dimethyl-PGF2beta and PGF2α highlights distinct biological activities:
Feature | 16,16-Dimethyl-PGF2beta | Prostaglandin F2alpha (PGF2α) |
---|---|---|
Receptor Affinity | Higher affinity for FP receptors | Binds to FP and EP receptors |
Radioprotective Effects | Significant protection against radiation | Limited radioprotective properties |
Stem Cell Activity | Enhances ESC hematopoietic formation | Less effect on stem cell differentiation |
Clinical Applications | Potential use in cancer therapy | Used in obstetrics and gynecology |
Case Studies
Case Study 1: Radioprotection in Mice
In a controlled study involving B6D2F1 mice, administration of 16,16-dimethyl-PGF2beta prior to exposure to ionizing radiation resulted in a significant increase in survival rates of intestinal stem cells. The study concluded that this compound could be a valuable agent for mitigating radiation damage during cancer treatments .
Case Study 2: Stem Cell Differentiation Enhancement
Another study focused on the effects of 16,16-dimethyl-PGF2beta on mouse embryonic stem cells showed increased colony formation capabilities when treated with this compound. This finding suggests potential applications in developing therapies for hematological disorders or enhancing tissue regeneration post-injury .
Propriétés
IUPAC Name |
(Z)-7-[(1R,2R,3R,5R)-3,5-dihydroxy-2-[(E,3R)-3-hydroxy-4,4-dimethyloct-1-enyl]cyclopentyl]hept-5-enoic acid | |
---|---|---|
Source | PubChem | |
URL | https://pubchem.ncbi.nlm.nih.gov | |
Description | Data deposited in or computed by PubChem | |
InChI |
InChI=1S/C22H38O5/c1-4-5-14-22(2,3)20(25)13-12-17-16(18(23)15-19(17)24)10-8-6-7-9-11-21(26)27/h6,8,12-13,16-20,23-25H,4-5,7,9-11,14-15H2,1-3H3,(H,26,27)/b8-6-,13-12+/t16-,17-,18-,19-,20-/m1/s1 | |
Source | PubChem | |
URL | https://pubchem.ncbi.nlm.nih.gov | |
Description | Data deposited in or computed by PubChem | |
InChI Key |
YMRWVEHSLXJOCD-OPVFONCOSA-N | |
Source | PubChem | |
URL | https://pubchem.ncbi.nlm.nih.gov | |
Description | Data deposited in or computed by PubChem | |
Canonical SMILES |
CCCCC(C)(C)C(C=CC1C(CC(C1CC=CCCCC(=O)O)O)O)O | |
Source | PubChem | |
URL | https://pubchem.ncbi.nlm.nih.gov | |
Description | Data deposited in or computed by PubChem | |
Isomeric SMILES |
CCCCC(C)(C)[C@@H](/C=C/[C@H]1[C@@H](C[C@H]([C@@H]1C/C=C\CCCC(=O)O)O)O)O | |
Source | PubChem | |
URL | https://pubchem.ncbi.nlm.nih.gov | |
Description | Data deposited in or computed by PubChem | |
Molecular Formula |
C22H38O5 | |
Source | PubChem | |
URL | https://pubchem.ncbi.nlm.nih.gov | |
Description | Data deposited in or computed by PubChem | |
Molecular Weight |
382.5 g/mol | |
Source | PubChem | |
URL | https://pubchem.ncbi.nlm.nih.gov | |
Description | Data deposited in or computed by PubChem | |
Retrosynthesis Analysis
AI-Powered Synthesis Planning: Our tool employs the Template_relevance Pistachio, Template_relevance Bkms_metabolic, Template_relevance Pistachio_ringbreaker, Template_relevance Reaxys, Template_relevance Reaxys_biocatalysis model, leveraging a vast database of chemical reactions to predict feasible synthetic routes.
One-Step Synthesis Focus: Specifically designed for one-step synthesis, it provides concise and direct routes for your target compounds, streamlining the synthesis process.
Accurate Predictions: Utilizing the extensive PISTACHIO, BKMS_METABOLIC, PISTACHIO_RINGBREAKER, REAXYS, REAXYS_BIOCATALYSIS database, our tool offers high-accuracy predictions, reflecting the latest in chemical research and data.
Strategy Settings
Precursor scoring | Relevance Heuristic |
---|---|
Min. plausibility | 0.01 |
Model | Template_relevance |
Template Set | Pistachio/Bkms_metabolic/Pistachio_ringbreaker/Reaxys/Reaxys_biocatalysis |
Top-N result to add to graph | 6 |
Feasible Synthetic Routes
Avertissement et informations sur les produits de recherche in vitro
Veuillez noter que tous les articles et informations sur les produits présentés sur BenchChem sont destinés uniquement à des fins informatives. Les produits disponibles à l'achat sur BenchChem sont spécifiquement conçus pour des études in vitro, qui sont réalisées en dehors des organismes vivants. Les études in vitro, dérivées du terme latin "in verre", impliquent des expériences réalisées dans des environnements de laboratoire contrôlés à l'aide de cellules ou de tissus. Il est important de noter que ces produits ne sont pas classés comme médicaments et n'ont pas reçu l'approbation de la FDA pour la prévention, le traitement ou la guérison de toute condition médicale, affection ou maladie. Nous devons souligner que toute forme d'introduction corporelle de ces produits chez les humains ou les animaux est strictement interdite par la loi. Il est essentiel de respecter ces directives pour assurer la conformité aux normes légales et éthiques en matière de recherche et d'expérimentation.