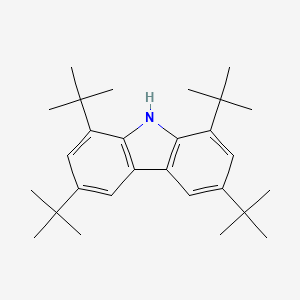
1,3,6,8-Tetratert-butyl-9H-carbazole
Vue d'ensemble
Description
1,3,6,8-Tetratert-butyl-9H-carbazole is a useful research compound. Its molecular formula is C28H41N and its molecular weight is 391.6 g/mol. The purity is usually 95%.
The exact mass of the compound this compound is unknown and the complexity rating of the compound is unknown. The United Nations designated GHS hazard class pictogram is Irritant, and the GHS signal word is WarningThe storage condition is unknown. Please store according to label instructions upon receipt of goods.
BenchChem offers high-quality this compound suitable for many research applications. Different packaging options are available to accommodate customers' requirements. Please inquire for more information about this compound including the price, delivery time, and more detailed information at info@benchchem.com.
Mécanisme D'action
Target of Action
Carbazole-based compounds are known for their excellent optoelectronic properties, high charge carrier mobility, and excellent morphological stability , which make them potential candidates in the field of nanodevices, rechargeable batteries, and electrochemical transistors .
Mode of Action
It is known that carbazole moieties can be electropolymerized in two different methods resulting in the formation of poly (2,7-carbazole)s or poly (3,6-carbazole)s derivatives . The fundamental difference between these two polymers is in the bandgap energies and effectual conjugation length .
Biochemical Pathways
It is known that carbazole-based compounds have intriguing properties including the presence of bridged biphenyl unit providing a material with a lower bandgap .
Pharmacokinetics
The compound has a molecular weight of 39164 . It is stored at a temperature of 28 C .
Result of Action
It is known that carbazole-based compounds have excellent electrical, electrochemical properties, good environmental stability, and unique optical properties .
Action Environment
It is known that carbazole-based compounds have good environmental stability .
Analyse Biochimique
Biochemical Properties
1,3,6,8-Tetratert-butyl-9H-carbazole plays a significant role in biochemical reactions due to its unique structure and properties. It interacts with various enzymes, proteins, and other biomolecules, influencing their activity and function. For instance, it has been observed to interact with the aryl hydrocarbon receptor (AhR), a protein that regulates the expression of various genes involved in detoxification processes. The interaction between this compound and AhR leads to the activation of the receptor, which in turn modulates the expression of genes involved in the metabolism of xenobiotics .
Cellular Effects
This compound has been shown to affect various types of cells and cellular processes. It influences cell function by modulating cell signaling pathways, gene expression, and cellular metabolism. For example, studies have demonstrated that this compound can activate the hypoxia-inducible factor-1 (HIF-1) pathway, leading to changes in gene expression and promoting angiogenesis in endothelial cells. Additionally, it has been found to affect the production of reactive oxygen species (ROS) in cells, which can impact cellular metabolism and energy production.
Molecular Mechanism
The molecular mechanism of action of this compound involves its interaction with various biomolecules and the modulation of their activity. One of the key mechanisms is its binding to the aryl hydrocarbon receptor (AhR), which leads to the activation of the receptor and subsequent changes in gene expression. This interaction can result in the upregulation of genes involved in detoxification processes and the metabolism of xenobiotics . Additionally, this compound has been shown to inhibit the activity of certain enzymes involved in energy metabolism, leading to changes in cellular energy production and metabolism.
Temporal Effects in Laboratory Settings
In laboratory settings, the effects of this compound have been observed to change over time. The compound is relatively stable, but it can undergo degradation under certain conditions, leading to changes in its activity and effects on cellular function. Long-term studies have shown that prolonged exposure to this compound can result in sustained changes in gene expression and cellular metabolism, which can impact cellular function and health.
Dosage Effects in Animal Models
The effects of this compound vary with different dosages in animal models. At low doses, the compound has been found to have minimal effects on cellular function and metabolism. At higher doses, it can lead to toxic effects, including increased production of reactive oxygen species (ROS) and disruption of energy metabolism. These effects can result in adverse outcomes, such as oxidative stress and damage to cellular structures.
Metabolic Pathways
This compound is involved in various metabolic pathways, including those related to the metabolism of xenobiotics and energy production. The compound interacts with enzymes and cofactors involved in these pathways, leading to changes in metabolic flux and metabolite levels. For example, it has been shown to affect the tricarboxylic acid (TCA) cycle, leading to alterations in energy metabolism and the production of adenosine triphosphate (ATP).
Transport and Distribution
Within cells and tissues, this compound is transported and distributed through interactions with various transporters and binding proteins. These interactions can influence the localization and accumulation of the compound within specific cellular compartments. For instance, it has been observed to accumulate in the endoplasmic reticulum and mitochondria, where it can exert its effects on cellular metabolism and energy production.
Subcellular Localization
The subcellular localization of this compound plays a crucial role in its activity and function. The compound is directed to specific compartments or organelles through targeting signals and post-translational modifications. For example, its accumulation in the mitochondria can lead to changes in mitochondrial function and energy production, while its presence in the endoplasmic reticulum can impact protein folding and detoxification processes.
Propriétés
IUPAC Name |
1,3,6,8-tetratert-butyl-9H-carbazole | |
---|---|---|
Source | PubChem | |
URL | https://pubchem.ncbi.nlm.nih.gov | |
Description | Data deposited in or computed by PubChem | |
InChI |
InChI=1S/C28H41N/c1-25(2,3)17-13-19-20-14-18(26(4,5)6)16-22(28(10,11)12)24(20)29-23(19)21(15-17)27(7,8)9/h13-16,29H,1-12H3 | |
Source | PubChem | |
URL | https://pubchem.ncbi.nlm.nih.gov | |
Description | Data deposited in or computed by PubChem | |
InChI Key |
OVSGNPWPCZRNKI-UHFFFAOYSA-N | |
Source | PubChem | |
URL | https://pubchem.ncbi.nlm.nih.gov | |
Description | Data deposited in or computed by PubChem | |
Canonical SMILES |
CC(C)(C)C1=CC2=C(C(=C1)C(C)(C)C)NC3=C2C=C(C=C3C(C)(C)C)C(C)(C)C | |
Source | PubChem | |
URL | https://pubchem.ncbi.nlm.nih.gov | |
Description | Data deposited in or computed by PubChem | |
Molecular Formula |
C28H41N | |
Source | PubChem | |
URL | https://pubchem.ncbi.nlm.nih.gov | |
Description | Data deposited in or computed by PubChem | |
DSSTOX Substance ID |
DTXSID60337905 | |
Record name | 1,3,6,8-Tetratert-butyl-9H-carbazole | |
Source | EPA DSSTox | |
URL | https://comptox.epa.gov/dashboard/DTXSID60337905 | |
Description | DSSTox provides a high quality public chemistry resource for supporting improved predictive toxicology. | |
Molecular Weight |
391.6 g/mol | |
Source | PubChem | |
URL | https://pubchem.ncbi.nlm.nih.gov | |
Description | Data deposited in or computed by PubChem | |
CAS No. |
34601-54-2 | |
Record name | 1,3,6,8-Tetratert-butyl-9H-carbazole | |
Source | EPA DSSTox | |
URL | https://comptox.epa.gov/dashboard/DTXSID60337905 | |
Description | DSSTox provides a high quality public chemistry resource for supporting improved predictive toxicology. | |
Record name | 1,3,6,8-Tetra-tert-butylcarbazole | |
Source | European Chemicals Agency (ECHA) | |
URL | https://echa.europa.eu/information-on-chemicals | |
Description | The European Chemicals Agency (ECHA) is an agency of the European Union which is the driving force among regulatory authorities in implementing the EU's groundbreaking chemicals legislation for the benefit of human health and the environment as well as for innovation and competitiveness. | |
Explanation | Use of the information, documents and data from the ECHA website is subject to the terms and conditions of this Legal Notice, and subject to other binding limitations provided for under applicable law, the information, documents and data made available on the ECHA website may be reproduced, distributed and/or used, totally or in part, for non-commercial purposes provided that ECHA is acknowledged as the source: "Source: European Chemicals Agency, http://echa.europa.eu/". Such acknowledgement must be included in each copy of the material. ECHA permits and encourages organisations and individuals to create links to the ECHA website under the following cumulative conditions: Links can only be made to webpages that provide a link to the Legal Notice page. | |
Retrosynthesis Analysis
AI-Powered Synthesis Planning: Our tool employs the Template_relevance Pistachio, Template_relevance Bkms_metabolic, Template_relevance Pistachio_ringbreaker, Template_relevance Reaxys, Template_relevance Reaxys_biocatalysis model, leveraging a vast database of chemical reactions to predict feasible synthetic routes.
One-Step Synthesis Focus: Specifically designed for one-step synthesis, it provides concise and direct routes for your target compounds, streamlining the synthesis process.
Accurate Predictions: Utilizing the extensive PISTACHIO, BKMS_METABOLIC, PISTACHIO_RINGBREAKER, REAXYS, REAXYS_BIOCATALYSIS database, our tool offers high-accuracy predictions, reflecting the latest in chemical research and data.
Strategy Settings
Precursor scoring | Relevance Heuristic |
---|---|
Min. plausibility | 0.01 |
Model | Template_relevance |
Template Set | Pistachio/Bkms_metabolic/Pistachio_ringbreaker/Reaxys/Reaxys_biocatalysis |
Top-N result to add to graph | 6 |
Feasible Synthetic Routes
Avertissement et informations sur les produits de recherche in vitro
Veuillez noter que tous les articles et informations sur les produits présentés sur BenchChem sont destinés uniquement à des fins informatives. Les produits disponibles à l'achat sur BenchChem sont spécifiquement conçus pour des études in vitro, qui sont réalisées en dehors des organismes vivants. Les études in vitro, dérivées du terme latin "in verre", impliquent des expériences réalisées dans des environnements de laboratoire contrôlés à l'aide de cellules ou de tissus. Il est important de noter que ces produits ne sont pas classés comme médicaments et n'ont pas reçu l'approbation de la FDA pour la prévention, le traitement ou la guérison de toute condition médicale, affection ou maladie. Nous devons souligner que toute forme d'introduction corporelle de ces produits chez les humains ou les animaux est strictement interdite par la loi. Il est essentiel de respecter ces directives pour assurer la conformité aux normes légales et éthiques en matière de recherche et d'expérimentation.