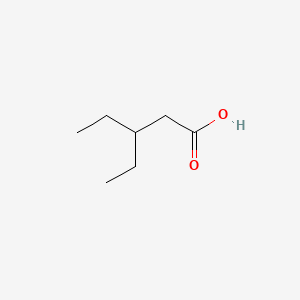
3-Ethylpentanoic acid
Vue d'ensemble
Description
3-Ethylpentanoic acid is a chemical compound with the molecular formula C7H14O2 . It has an average mass of 130.185 Da and a monoisotopic mass of 130.099380 Da .
Molecular Structure Analysis
The 3-Ethylpentanoic acid molecule contains a total of 22 bonds. There are 8 non-H bonds, 1 multiple bond, 4 rotatable bonds, 1 double bond, 1 carboxylic acid (aliphatic), and 1 hydroxyl group .Physical And Chemical Properties Analysis
3-Ethylpentanoic acid is a chemical compound with the molecular formula C7H14O2 . It has an average mass of 130.185 Da and a monoisotopic mass of 130.099380 Da .Applications De Recherche Scientifique
Organic Synthesis and Chemical Reactions
- 3-Ethylpentanoic acid and its derivatives are involved in various chemical reactions and synthesis processes. For example, the preparation of 3-hydroxy-3-methyl-5-phenylpentanoic acid involves the reflux of ethyl 3-hydroxy-3-methyl-5-phenylpentanoate with potassium hydroxide, indicating a role in synthesizing complex organic compounds (Stoermer & Pinhey, 1998).
- The compound also finds application in the synthesis of other organic molecules, like 3-Methylpentanoic acid, showcasing its utility in diverse organic syntheses (Vliet, Marvel, & Hsueh, 2003).
Plant and Insect Research
- In the field of botany and entomology, derivatives of 3-Ethylpentanoic acid, such as 3-pentanol, have been shown to prime plant immunity against bacterial pathogens like Pseudomonas syringae in Arabidopsis, indicating its potential in agricultural biotechnology [(Song, Choi, & Ryu, 2015)](https://consensus.app/papers/3pentanol-primes-plant-immunity-speck-pathogen-song/021e603866495db99453062ffdcaf223/?utm_source=chatgpt).
- Furthermore, 3-ethyl-4-methylpentanol, a closely related compound, plays a crucial role in the mating behavior of certain ant species, suggesting its importance in chemical communication within the insect world (Castracani et al., 2008).
Medicinal Chemistry
- Ethyl-eicosapentanoic acid, a derivative, has been studied for its neuroprotective effects in bipolar disorder, highlighting the potential medicinal applications of compounds derived from 3-Ethylpentanoic acid in neuropsychiatric disorders (Frangou, Lewis, Wollard, & Simmons, 2007).
Environmental Science
- In environmental science, derivatives of 3-Ethylpentanoic acid are identified in atmospheric studies. For instance, certain aerosol compounds that are products of secondary organic aerosol from monoterpenes were found in both field and laboratory samples, suggesting a role in atmospheric chemistry and environmental monitoring (Jaoui et al., 2005).
Propriétés
IUPAC Name |
3-ethylpentanoic acid | |
---|---|---|
Source | PubChem | |
URL | https://pubchem.ncbi.nlm.nih.gov | |
Description | Data deposited in or computed by PubChem | |
InChI |
InChI=1S/C7H14O2/c1-3-6(4-2)5-7(8)9/h6H,3-5H2,1-2H3,(H,8,9) | |
Source | PubChem | |
URL | https://pubchem.ncbi.nlm.nih.gov | |
Description | Data deposited in or computed by PubChem | |
InChI Key |
ATUUSOSLBXVJKL-UHFFFAOYSA-N | |
Source | PubChem | |
URL | https://pubchem.ncbi.nlm.nih.gov | |
Description | Data deposited in or computed by PubChem | |
Canonical SMILES |
CCC(CC)CC(=O)O | |
Source | PubChem | |
URL | https://pubchem.ncbi.nlm.nih.gov | |
Description | Data deposited in or computed by PubChem | |
Molecular Formula |
C7H14O2 | |
Source | PubChem | |
URL | https://pubchem.ncbi.nlm.nih.gov | |
Description | Data deposited in or computed by PubChem | |
DSSTOX Substance ID |
DTXSID20207594 | |
Record name | Pentanoic acid, 3-ethyl- | |
Source | EPA DSSTox | |
URL | https://comptox.epa.gov/dashboard/DTXSID20207594 | |
Description | DSSTox provides a high quality public chemistry resource for supporting improved predictive toxicology. | |
Molecular Weight |
130.18 g/mol | |
Source | PubChem | |
URL | https://pubchem.ncbi.nlm.nih.gov | |
Description | Data deposited in or computed by PubChem | |
CAS RN |
58888-87-2 | |
Record name | 3-Ethylpentanoic acid | |
Source | CAS Common Chemistry | |
URL | https://commonchemistry.cas.org/detail?cas_rn=58888-87-2 | |
Description | CAS Common Chemistry is an open community resource for accessing chemical information. Nearly 500,000 chemical substances from CAS REGISTRY cover areas of community interest, including common and frequently regulated chemicals, and those relevant to high school and undergraduate chemistry classes. This chemical information, curated by our expert scientists, is provided in alignment with our mission as a division of the American Chemical Society. | |
Explanation | The data from CAS Common Chemistry is provided under a CC-BY-NC 4.0 license, unless otherwise stated. | |
Record name | Pentanoic acid, 3-ethyl- | |
Source | ChemIDplus | |
URL | https://pubchem.ncbi.nlm.nih.gov/substance/?source=chemidplus&sourceid=0058888872 | |
Description | ChemIDplus is a free, web search system that provides access to the structure and nomenclature authority files used for the identification of chemical substances cited in National Library of Medicine (NLM) databases, including the TOXNET system. | |
Record name | Pentanoic acid, 3-ethyl- | |
Source | EPA DSSTox | |
URL | https://comptox.epa.gov/dashboard/DTXSID20207594 | |
Description | DSSTox provides a high quality public chemistry resource for supporting improved predictive toxicology. | |
Synthesis routes and methods I
Procedure details
Synthesis routes and methods II
Procedure details
Retrosynthesis Analysis
AI-Powered Synthesis Planning: Our tool employs the Template_relevance Pistachio, Template_relevance Bkms_metabolic, Template_relevance Pistachio_ringbreaker, Template_relevance Reaxys, Template_relevance Reaxys_biocatalysis model, leveraging a vast database of chemical reactions to predict feasible synthetic routes.
One-Step Synthesis Focus: Specifically designed for one-step synthesis, it provides concise and direct routes for your target compounds, streamlining the synthesis process.
Accurate Predictions: Utilizing the extensive PISTACHIO, BKMS_METABOLIC, PISTACHIO_RINGBREAKER, REAXYS, REAXYS_BIOCATALYSIS database, our tool offers high-accuracy predictions, reflecting the latest in chemical research and data.
Strategy Settings
Precursor scoring | Relevance Heuristic |
---|---|
Min. plausibility | 0.01 |
Model | Template_relevance |
Template Set | Pistachio/Bkms_metabolic/Pistachio_ringbreaker/Reaxys/Reaxys_biocatalysis |
Top-N result to add to graph | 6 |
Feasible Synthetic Routes
Avertissement et informations sur les produits de recherche in vitro
Veuillez noter que tous les articles et informations sur les produits présentés sur BenchChem sont destinés uniquement à des fins informatives. Les produits disponibles à l'achat sur BenchChem sont spécifiquement conçus pour des études in vitro, qui sont réalisées en dehors des organismes vivants. Les études in vitro, dérivées du terme latin "in verre", impliquent des expériences réalisées dans des environnements de laboratoire contrôlés à l'aide de cellules ou de tissus. Il est important de noter que ces produits ne sont pas classés comme médicaments et n'ont pas reçu l'approbation de la FDA pour la prévention, le traitement ou la guérison de toute condition médicale, affection ou maladie. Nous devons souligner que toute forme d'introduction corporelle de ces produits chez les humains ou les animaux est strictement interdite par la loi. Il est essentiel de respecter ces directives pour assurer la conformité aux normes légales et éthiques en matière de recherche et d'expérimentation.