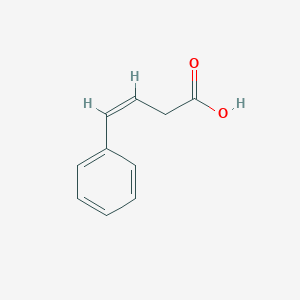
trans-Styrylacetic acid
Vue d'ensemble
Description
trans-Styrylacetic acid (IUPAC name: (3E)-4-phenyl-3-butenoic acid) is an α,β-unsaturated carboxylic acid with the molecular formula C₁₀H₁₀O₂ and a molecular weight of 162.19 g/mol. Its structure features a trans-conjugated double bond (C3–C4), a phenyl group at C4, and a carboxylic acid moiety at C1 (Fig. 1). The compound is characterized by a melting point of 84–86°C and exhibits hydrogen-bonding interactions in its crystalline form, as demonstrated by polarized IR spectroscopy . It serves as a versatile intermediate in organic synthesis, particularly in Pd-catalyzed α-arylation , esterification , and amide formation .
Méthodes De Préparation
Wittig Reaction: A Robust Pathway for trans-Styrylacetic Acid Synthesis
The Wittig reaction is the most widely reported method for synthesizing this compound. This approach leverages the reactivity of ylides with carbonyl compounds to form alkenes with high stereoselectivity. The general procedure involves the reaction of (2-carboxyethyl)triphenylphosphonium bromide with substituted benzaldehydes under strongly basic conditions .
Reaction Mechanism and Stereochemical Control
The reaction proceeds via the generation of a phosphorus ylide from (2-carboxyethyl)triphenylphosphonium bromide using a strong base such as potassium tert-butoxide (KOt-Bu). The ylide then reacts with the benzaldehyde derivative to form the trans-alkene product. The stereoselectivity arises from the anti-periplanar geometry of the ylide and aldehyde during the transition state, favoring the trans-configuration .
Experimental Procedure and Optimization
A representative synthesis from the literature involves the following steps :
-
Reagent Preparation : (2-Carboxyethyl)triphenylphosphonium bromide (6 mmol) and substituted benzaldehyde (9 mmol) are dissolved in anhydrous tetrahydrofuran (THF).
-
Base Addition : Potassium tert-butoxide (13 mmol) is added dropwise at 0°C to generate the ylide.
-
Reaction Workup : After stirring overnight at room temperature, the mixture is acidified with 6 M HCl to pH 1. The aqueous layer is extracted with diethyl ether, and the combined organic phases are washed with saturated sodium bicarbonate and brine.
-
Purification : The crude product is purified via flash chromatography (hexane/ethyl acetate), yielding this compound derivatives in 70–74% yield .
Alternative Synthetic Routes and Comparative Analysis
While the Wittig reaction dominates the literature, other methods have been explored for specialized applications:
Critical Factors in Reaction Optimization
Base Selection and Solvent Effects
The choice of base significantly impacts ylide formation. Potassium tert-butoxide is preferred over alternatives like lithium diisopropylamide (LDA) due to its ease of handling and compatibility with THF . Polar aprotic solvents (e.g., THF, acetonitrile) enhance ylide stability and reaction homogeneity .
Temperature and Reaction Time
Optimal yields are achieved at 0°C to room temperature with overnight stirring. Prolonged heating risks side reactions such as decarboxylation or isomerization .
Purification Challenges
The carboxylic acid functionality necessitates careful pH control during extraction to prevent emulsion formation. Flash chromatography with hexane/ethyl acetate (7:3) effectively separates the product from triphenylphosphine oxide byproducts .
Applications in Natural Product Synthesis
This compound serves as a key building block in the synthesis of cryptophycin analogs, a class of antimitotic agents. For example, Shi epoxidation of this compound followed by lactonization yields δ-hydroxy acid precursors for cryptophycin-39 . The acid’s conjugated system also facilitates [4+2] cycloadditions in quinolinone and chromanone synthesis .
Analyse Des Réactions Chimiques
Types of Reactions:
Oxidation: trans-Styrylacetic acid can undergo oxidation reactions to form various oxidized products.
Substitution: this compound can participate in substitution reactions, particularly at the phenyl ring.
Common Reagents and Conditions:
Oxidation: Potassium permanganate in aqueous solution, chromium trioxide in acetic acid.
Reduction: Lithium aluminum hydride in ether, sodium borohydride in methanol.
Substitution: Electrophilic reagents such as bromine or nitric acid in the presence of a catalyst.
Major Products:
Oxidation: Formation of carboxylic acids or ketones.
Reduction: Formation of alcohols.
Substitution: Formation of substituted phenyl derivatives.
Applications De Recherche Scientifique
Mechanism-Based Inhibition
trans-Styrylacetic acid acts as a mechanism-based inhibitor for Peptidylglycine α-hydroxylating monooxygenase. This enzyme plays a crucial role in the post-translational modification of neuropeptides, making this compound valuable in studying neuropeptide biosynthesis.
Cancer Research
Research indicates that this compound exhibits tumorigenic properties by modulating key signaling pathways involved in cancer cell proliferation and apoptosis. Notably, it influences pathways such as p38 MAPK and JNK, showing selective effects on tumorigenic cells compared to non-tumorigenic cells .
Case Study:
- A comparative study demonstrated that this compound and vorinostat (a known histone deacetylase inhibitor) both inhibited growth in tumorigenic cell lines while sparing normal cells, suggesting its potential as a selective anti-cancer agent .
Microbial Degradation
This compound is integral to the microbial degradation of styrene, an environmental pollutant. Various microorganisms can metabolize styrene aerobically and anaerobically, transforming it into less harmful compounds such as phenylacetic acid and benzoic acid. This catabolic pathway is essential for bioremediation efforts targeting aromatic pollutants .
Organic Synthesis
In organic chemistry, this compound serves as a versatile building block for synthesizing various organic compounds. Its structural features allow it to participate in multiple chemical reactions, facilitating the development of complex molecules used in pharmaceuticals and agrochemicals .
Environmental Applications
The compound's role in the degradation of environmental pollutants underscores its importance in environmental microbiology. By participating in the phenylacetyl-CoA catabolism pathway, this compound contributes to the bioremediation processes that detoxify contaminated environments .
Summary Table of Applications
Application Area | Specific Use | Key Findings/Outcomes |
---|---|---|
Biochemistry | Inhibitor of Peptidylglycine α-hydroxylating monooxygenase | Modulates neuropeptide biosynthesis |
Cancer Research | Tumorigenic pathway modulation | Selective inhibition of tumor cell growth |
Microbial Degradation | Degradation of styrene | Contributes to bioremediation by transforming pollutants |
Organic Synthesis | Building block for organic compounds | Facilitates complex molecule synthesis |
Environmental Microbiology | Catabolic pathway for aromatic compounds | Essential for detoxifying environments |
Mécanisme D'action
The mechanism of action of trans-Styrylacetic acid involves its interaction with specific molecular targets, such as enzymes. For instance, as an inhibitor of Peptidylglycine α-hydroxylating monooxygenase, this compound binds to the active site of the enzyme, preventing it from catalyzing its normal substrate. This inhibition can lead to downstream effects on peptide modification and biological activity .
Comparaison Avec Des Composés Similaires
Comparison with Structurally Similar Compounds
Geometric Isomer: cis-Styrylacetic Acid
While trans-Styrylacetic acid is widely studied, its cis-isomer is less common in synthetic applications. The trans configuration stabilizes the molecule through conjugation between the double bond and the carboxylic acid group, enhancing resonance effects. In contrast, the cis isomer may exhibit steric hindrance between the phenyl group and the carboxylic acid, reducing stability and reactivity. No direct data on cis-styrylacetic acid’s properties are provided in the evidence, but geometric isomerism generally influences melting points and solubility due to packing efficiency differences in crystals.
Vinylphenylacetic Acid
Vinylphenylacetic acid (a structural isomer with the double bond between C2–C3) is noted to form decarboxylated side products during reactions, unlike this compound, which avoids such side reactions under similar conditions . This highlights the superior synthetic utility of this compound in avoiding undesired pathways.
Cinnamic Acid (3-Phenylpropenoic Acid)
Cinnamic acid (C₉H₈O₂, MW = 148.16 g/mol) shares a conjugated trans double bond and carboxylic acid group but lacks the extended carbon chain of this compound (Table 1). Key differences include:
- Melting Point : Cinnamic acid melts at 133°C , significantly higher than this compound, likely due to tighter crystal packing .
- Applications : Cinnamic acid is widely used in food preservation and polymer synthesis, whereas this compound is primarily employed in medicinal chemistry (e.g., anti-leishmanial agent synthesis) .
4-(4-Fluorophenyl)-4-phenylbut-3-enoic Acid (4ad)
This fluorinated derivative, synthesized via Pd-catalyzed α-arylation of this compound , demonstrates how substituents on the phenyl ring modulate bioactivity.
Comparative Data Tables
Table 1: Physical Properties of this compound and Analogues
Compound | Molecular Formula | Molecular Weight (g/mol) | Melting Point (°C) | Key Functional Groups |
---|---|---|---|---|
This compound | C₁₀H₁₀O₂ | 162.19 | 84–86 | Carboxylic acid, trans double bond |
Cinnamic acid | C₉H₈O₂ | 148.16 | 133 | Carboxylic acid, trans double bond |
Vinylphenylacetic acid* | C₁₀H₁₀O₂ | 162.19 | N/A | Carboxylic acid, vinyl group |
4ad (fluorophenyl derivative) | C₁₆H₁₃FO₂ | 264.27 | N/A | Carboxylic acid, fluorophenyl group |
Green Chemistry Considerations
This compound is highlighted as a green alternative with high atom economy (96% purity) and energy-efficient synthesis protocols . Its use of renewable feedstocks contrasts with traditional methods for similar compounds, which may involve hazardous reagents or lower yields.
Activité Biologique
Trans-Styrylacetic acid (4-Phenyl-3-butenoic acid) is a compound that has garnered attention for its diverse biological activities, particularly in the fields of cancer research, microbiology, and organic synthesis. This article reviews the current understanding of its biological properties, focusing on its mechanisms of action, effects on cellular pathways, and potential therapeutic applications.
This compound is characterized by its unique structure, which includes a trans double bond configuration. Its physical properties include:
- Molecular Formula : C₁₄H₁₂O₂
- Density : 1.145 g/cm³
- Boiling Point : 302 °C
- Flash Point : 215.8 °C
- Solubility : Soluble in organic solvents with moderate hydrophobicity (ACD/LogP value of 2.18) .
Cancer Research
This compound has been identified as a potential tumorigenic agent, exhibiting selective modulation of signaling pathways associated with cancer cell proliferation. Research indicates that it influences key pathways such as:
- p38 MAPK : Involved in stress responses and cell differentiation.
- JNK (c-Jun N-terminal kinase) : Plays a critical role in apoptosis and cellular stress responses.
Studies have shown that this compound can inhibit the growth of tumorigenic cell lines while sparing non-tumorigenic cells, suggesting a selective cytotoxicity that could be harnessed for therapeutic purposes .
Microbial Degradation
In environmental microbiology, this compound is involved in the microbial degradation of styrene, a common pollutant. Various microorganisms can metabolize styrene under aerobic and anaerobic conditions, yielding products like phenylacetic acid and benzoic acid. This process contributes to bioremediation efforts aimed at reducing environmental pollutants .
In Vitro Studies on Tumorigenic Cells
A comparative study evaluated the effects of this compound against Vorinostat (a known histone deacetylase inhibitor) on various tumorigenic cell lines. The results indicated:
- Both compounds significantly inhibited the growth of H2009 human lung carcinoma cells.
- This compound increased phosphorylation of p38 MAPK in tumorigenic cells but did not affect non-tumorigenic cells significantly.
- Selectivity for tumorigenic cells was evident, with reduced effects observed in non-tumorigenic models .
Compound | Effect on Tumorigenic Cells | Effect on Non-Tumorigenic Cells |
---|---|---|
This compound | Significant inhibition | Minimal effect |
Vorinostat | Significant inhibition | Significant inhibition |
Microbial Catabolism Studies
Research has demonstrated that this compound can be catabolized by Pseudomonas putida, highlighting its role in the phenylacetyl-CoA catabolon. This pathway is crucial for microbial degradation processes and showcases the compound's potential in biotechnological applications .
Q & A
Basic Research Questions
Q. What are the standard synthetic protocols for preparing trans-styrylacetic acid, and how can purity be verified?
this compound is typically synthesized via a two-step process involving activation of the carboxylic acid group. For example, a THF-based reaction with carbonyldiimidazole (CDI) facilitates intermediate formation, followed by coupling with MeONHMe·HCl in the presence of NaH to yield the Weinreb amide derivative . Purity is assessed using analytical techniques such as:
- ¹H/¹³C NMR to confirm structural integrity (e.g., δ 7.38–7.20 ppm for aromatic protons).
- FT-IR for functional group analysis (e.g., peaks at 1749 cm⁻¹ for carbonyl stretches).
- HRMS to validate molecular weight (e.g., experimental m/z 206.1170 vs. calculated 206.1176 for C₁₂H₁₆NO₂) .
- Melting point determination (lit. range 82–88°C) to identify impurities .
Q. How should researchers handle and store this compound to ensure stability?
The compound is sensitive to temperature and moisture. Key storage guidelines include:
- Storing at 0–6°C in airtight containers to prevent degradation .
- Avoiding prolonged exposure to light or humidity, which may promote polymerization or side reactions .
Q. What analytical methods are recommended for characterizing this compound?
- Chromatography : Purification via flash chromatography (e.g., 80:20 hexanes/EtOAC) to isolate high-purity fractions .
- Spectroscopy : Combined NMR, FT-IR, and HRMS for structural confirmation .
- Thermal analysis : Differential scanning calorimetry (DSC) to resolve discrepancies in reported melting points (e.g., 82–88°C vs. 84–86°C) by assessing crystallinity and polymorphic forms .
Advanced Research Questions
Q. How can contradictions in reported physical properties (e.g., melting points) be systematically addressed?
Discrepancies may arise from variations in synthetic routes, purification methods, or impurities. To resolve these:
- Reproduce synthesis : Follow documented protocols (e.g., CDI-mediated activation in THF) .
- Control crystallization : Recrystallize using solvents like EtOAc/hexanes under controlled cooling rates to isolate pure polymorphs .
- Characterize impurities : Use LC-MS or HPLC to identify byproducts (e.g., unreacted intermediates) that alter thermal properties .
Q. What green chemistry principles can be applied to optimize this compound synthesis?
The compound’s "greener alternative score" (DOZN™) highlights opportunities for sustainability:
- Atom economy : Optimize stoichiometry to reduce waste (e.g., CDI coupling efficiency >90%) .
- Renewable solvents : Replace THF with cyclopentyl methyl ether (CPME) or 2-MeTHF, which have lower environmental toxicity .
- Energy efficiency : Use microwave-assisted synthesis to accelerate reaction times and reduce energy consumption .
Q. How can computational modeling aid in predicting this compound reactivity?
- DFT calculations : Model the electronic structure to predict sites for electrophilic/nucleophilic attack (e.g., α,β-unsaturated carbonyl system) .
- Molecular docking : Study interactions with biological targets (e.g., enzymes inhibited by styryl-based analogs) to guide drug discovery applications .
- Solubility parameters : Use COSMO-RS simulations to optimize solvent selection for reactions or formulations .
Q. What experimental design considerations are critical for reproducibility in this compound studies?
- Detailed protocols : Document reaction conditions (e.g., molar ratios, temperature gradients) to minimize variability .
- Blind testing : Validate analytical results across multiple labs to address bias .
- Negative controls : Include experiments without catalysts/reagents to identify side reactions .
Q. Methodological Guidelines
- Synthetic reproducibility : Always cross-reference CAS numbers (1914-58-5 vs. 2243-53-0) to confirm isomer identity, as misidentification can skew results .
- Data interpretation : Use statistical tools (e.g., ANOVA) to assess significance of melting point variations or reaction yields .
- Safety protocols : Adhere to hazard classifications (e.g., R36/37/38 for irritation risks) and use PPE during handling .
Propriétés
Numéro CAS |
1914-58-5 |
---|---|
Formule moléculaire |
C10H10O2 |
Poids moléculaire |
162.18 g/mol |
Nom IUPAC |
(Z)-4-phenylbut-3-enoic acid |
InChI |
InChI=1S/C10H10O2/c11-10(12)8-4-7-9-5-2-1-3-6-9/h1-7H,8H2,(H,11,12)/b7-4- |
Clé InChI |
PSCXFXNEYIHJST-DAXSKMNVSA-N |
SMILES |
C1=CC=C(C=C1)C=CCC(=O)O |
SMILES isomérique |
C1=CC=C(C=C1)/C=C\CC(=O)O |
SMILES canonique |
C1=CC=C(C=C1)C=CCC(=O)O |
Key on ui other cas no. |
59744-46-6 1914-58-5 |
Pictogrammes |
Irritant |
Synonymes |
4-phenyl-3-butenoic acid 4-phenyl-3-butenoic acid, (E)-isomer styrylacetic acid |
Origine du produit |
United States |
Retrosynthesis Analysis
AI-Powered Synthesis Planning: Our tool employs the Template_relevance Pistachio, Template_relevance Bkms_metabolic, Template_relevance Pistachio_ringbreaker, Template_relevance Reaxys, Template_relevance Reaxys_biocatalysis model, leveraging a vast database of chemical reactions to predict feasible synthetic routes.
One-Step Synthesis Focus: Specifically designed for one-step synthesis, it provides concise and direct routes for your target compounds, streamlining the synthesis process.
Accurate Predictions: Utilizing the extensive PISTACHIO, BKMS_METABOLIC, PISTACHIO_RINGBREAKER, REAXYS, REAXYS_BIOCATALYSIS database, our tool offers high-accuracy predictions, reflecting the latest in chemical research and data.
Strategy Settings
Precursor scoring | Relevance Heuristic |
---|---|
Min. plausibility | 0.01 |
Model | Template_relevance |
Template Set | Pistachio/Bkms_metabolic/Pistachio_ringbreaker/Reaxys/Reaxys_biocatalysis |
Top-N result to add to graph | 6 |
Feasible Synthetic Routes
Avertissement et informations sur les produits de recherche in vitro
Veuillez noter que tous les articles et informations sur les produits présentés sur BenchChem sont destinés uniquement à des fins informatives. Les produits disponibles à l'achat sur BenchChem sont spécifiquement conçus pour des études in vitro, qui sont réalisées en dehors des organismes vivants. Les études in vitro, dérivées du terme latin "in verre", impliquent des expériences réalisées dans des environnements de laboratoire contrôlés à l'aide de cellules ou de tissus. Il est important de noter que ces produits ne sont pas classés comme médicaments et n'ont pas reçu l'approbation de la FDA pour la prévention, le traitement ou la guérison de toute condition médicale, affection ou maladie. Nous devons souligner que toute forme d'introduction corporelle de ces produits chez les humains ou les animaux est strictement interdite par la loi. Il est essentiel de respecter ces directives pour assurer la conformité aux normes légales et éthiques en matière de recherche et d'expérimentation.