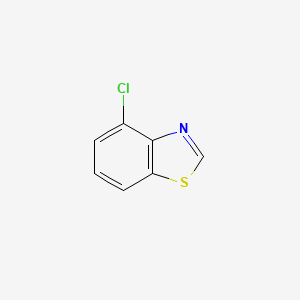
4-Chlorobenzothiazole
Vue d'ensemble
Description
4-Chlorobenzothiazole is an aromatic heterocyclic compound that consists of a benzene ring fused to a thiazole ring with a chlorine atom attached to the benzene ring. This compound is known for its significant role in various chemical and industrial applications due to its unique structural properties and reactivity.
Mécanisme D'action
Target of Action
4-Chlorobenzothiazole is a derivative of benzothiazole, a class of compounds known for their extensive biological activities Benzothiazoles are known to interact with a variety of enzymes and receptors, contributing to their diverse pharmacological effects .
Mode of Action
For instance, they can form complexes with targeted enzymes such as DNA gyrase and tyrosine kinase . The presence of the chlorine atom in this compound may influence its interaction with these targets, potentially enhancing its binding affinity or altering its mode of action.
Biochemical Pathways
Benzothiazoles are known to impact a wide range of biological pathways due to their interaction with various enzymes and receptors . For example, some benzothiazoles have been found to inhibit BCL-2, a key enzyme involved in apoptosis . This suggests that this compound could potentially influence pathways related to cell death and survival.
Pharmacokinetics
The pharmacokinetics of benzothiazole derivatives can vary widely depending on their specific chemical structure
Result of Action
Benzothiazoles are known to have a wide range of biological activities, including anti-cancer, anti-bacterial, anti-tuberculosis, anti-diabetic, and anti-inflammatory effects . These effects are likely the result of their interaction with various cellular targets and their influence on different biochemical pathways.
Analyse Biochimique
Biochemical Properties
4-Chlorobenzothiazole plays a crucial role in several biochemical reactions. It interacts with a variety of enzymes, proteins, and other biomolecules. For instance, it has been shown to inhibit the activity of the ubiquitin-activating enzyme (E1), which is involved in the ubiquitin-proteasome pathway . This interaction is significant because the ubiquitin-proteasome pathway is essential for protein degradation and regulation within the cell. Additionally, this compound has been found to interact with inflammatory factors such as interleukin-6 (IL-6) and tumor necrosis factor-alpha (TNF-α), reducing their activity and thereby exhibiting anti-inflammatory properties .
Cellular Effects
The effects of this compound on various types of cells and cellular processes are profound. In cancer cells, such as human epidermoid carcinoma (A431) and non-small cell lung cancer cells (A549), this compound inhibits cell proliferation and induces apoptosis . This compound also affects cell signaling pathways, including those involved in inflammation and cell migration. For example, it decreases the activity of IL-6 and TNF-α in mouse monocyte macrophages (RAW264.7), which are key players in the inflammatory response . Furthermore, this compound influences gene expression by modulating the levels of specific proteins involved in cell cycle regulation and apoptosis .
Molecular Mechanism
At the molecular level, this compound exerts its effects through several mechanisms. It binds to specific biomolecules, such as the ubiquitin-activating enzyme (E1), inhibiting its activity and thereby disrupting the ubiquitin-proteasome pathway . This inhibition leads to the accumulation of proteins that would otherwise be degraded, affecting various cellular processes. Additionally, this compound modulates gene expression by influencing the transcription and translation of genes involved in inflammation and cell cycle regulation . These molecular interactions highlight the compound’s potential as a therapeutic agent.
Temporal Effects in Laboratory Settings
In laboratory settings, the effects of this compound change over time. The compound’s stability and degradation are critical factors that influence its long-term effects on cellular function. Studies have shown that this compound remains stable under specific conditions, allowing for prolonged exposure in in vitro and in vivo experiments . Its degradation products can also have biological activities, which need to be considered when interpreting experimental results. Long-term studies have demonstrated that continuous exposure to this compound can lead to sustained inhibition of cell proliferation and induction of apoptosis in cancer cells .
Dosage Effects in Animal Models
The effects of this compound vary with different dosages in animal models. At lower doses, the compound exhibits significant anticancer and anti-inflammatory activities without causing adverse effects . At higher doses, toxic effects can be observed, including damage to vital organs and disruption of normal cellular functions . These threshold effects highlight the importance of determining the optimal dosage for therapeutic applications. Animal studies have provided valuable insights into the safe and effective use of this compound in potential treatments.
Metabolic Pathways
This compound is involved in several metabolic pathways within the cell. It interacts with enzymes such as cytochrome P450, which plays a crucial role in the metabolism of xenobiotics . The compound’s metabolism leads to the formation of various metabolites, some of which retain biological activity. These metabolic processes can influence the overall efficacy and toxicity of this compound. Understanding these pathways is essential for developing strategies to optimize its therapeutic potential.
Transport and Distribution
The transport and distribution of this compound within cells and tissues are mediated by specific transporters and binding proteins. For instance, it has been shown to interact with membrane transporters that facilitate its uptake into cells . Once inside the cell, this compound can accumulate in specific compartments, influencing its localization and activity. The distribution of the compound within tissues also affects its overall pharmacokinetics and pharmacodynamics, which are critical for its therapeutic application.
Subcellular Localization
This compound exhibits specific subcellular localization patterns that influence its activity and function. It can be targeted to various cellular compartments, including the cytoplasm, nucleus, and mitochondria . This localization is often mediated by targeting signals or post-translational modifications that direct the compound to specific organelles. The subcellular distribution of this compound can affect its interactions with biomolecules and its overall biological activity. Understanding these localization patterns is crucial for elucidating the compound’s mechanism of action.
Méthodes De Préparation
Synthetic Routes and Reaction Conditions: 4-Chlorobenzothiazole can be synthesized through several methods. One common method involves the cyclization of 2-aminobenzenethiol with a chlorinating agent. The reaction typically proceeds under acidic conditions, where the 2-aminobenzenethiol reacts with a chlorinating agent such as thionyl chloride or phosphorus pentachloride to form this compound .
Industrial Production Methods: In industrial settings, the production of this compound often involves the use of continuous flow reactors to ensure efficient and scalable synthesis. The process may include the use of catalysts to enhance the reaction rate and yield. Green chemistry principles are also applied to minimize the use of toxic solvents and reduce waste .
Analyse Des Réactions Chimiques
Types of Reactions: 4-Chlorobenzothiazole undergoes various chemical reactions, including:
Substitution Reactions: The chlorine atom can be substituted by nucleophiles such as amines, thiols, or alkoxides.
Oxidation Reactions: The thiazole ring can be oxidized to form sulfoxides or sulfones.
Reduction Reactions: The compound can be reduced to form 4-chlorobenzothiazoline
Common Reagents and Conditions:
Substitution Reactions: Reagents such as sodium amide or potassium thiolate are commonly used under basic conditions.
Oxidation Reactions: Oxidizing agents like hydrogen peroxide or m-chloroperbenzoic acid are used.
Reduction Reactions: Reducing agents such as lithium aluminum hydride or sodium borohydride are employed
Major Products:
Substitution Reactions: Products include various substituted benzothiazoles.
Oxidation Reactions: Products include sulfoxides and sulfones.
Reduction Reactions: Products include 4-chlorobenzothiazoline
Applications De Recherche Scientifique
4-Chlorobenzothiazole has a wide range of applications in scientific research:
Comparaison Avec Des Composés Similaires
Benzothiazole: Lacks the chlorine substituent and has different reactivity and applications.
2-Aminobenzothiazole: Contains an amino group instead of chlorine, leading to different chemical behavior and uses.
2-Mercaptobenzothiazole: Contains a thiol group, making it more reactive in certain types of chemical reactions
Uniqueness of 4-Chlorobenzothiazole: this compound is unique due to the presence of the chlorine atom, which enhances its reactivity in substitution reactions and provides distinct biological activities compared to its analogs .
Propriétés
IUPAC Name |
4-chloro-1,3-benzothiazole | |
---|---|---|
Source | PubChem | |
URL | https://pubchem.ncbi.nlm.nih.gov | |
Description | Data deposited in or computed by PubChem | |
InChI |
InChI=1S/C7H4ClNS/c8-5-2-1-3-6-7(5)9-4-10-6/h1-4H | |
Source | PubChem | |
URL | https://pubchem.ncbi.nlm.nih.gov | |
Description | Data deposited in or computed by PubChem | |
InChI Key |
IFEPGHPDQJOYGG-UHFFFAOYSA-N | |
Source | PubChem | |
URL | https://pubchem.ncbi.nlm.nih.gov | |
Description | Data deposited in or computed by PubChem | |
Canonical SMILES |
C1=CC2=C(C(=C1)Cl)N=CS2 | |
Source | PubChem | |
URL | https://pubchem.ncbi.nlm.nih.gov | |
Description | Data deposited in or computed by PubChem | |
Molecular Formula |
C7H4ClNS | |
Source | PubChem | |
URL | https://pubchem.ncbi.nlm.nih.gov | |
Description | Data deposited in or computed by PubChem | |
DSSTOX Substance ID |
DTXSID70445303 | |
Record name | 4-chlorobenzothiazole | |
Source | EPA DSSTox | |
URL | https://comptox.epa.gov/dashboard/DTXSID70445303 | |
Description | DSSTox provides a high quality public chemistry resource for supporting improved predictive toxicology. | |
Molecular Weight |
169.63 g/mol | |
Source | PubChem | |
URL | https://pubchem.ncbi.nlm.nih.gov | |
Description | Data deposited in or computed by PubChem | |
CAS No. |
3048-45-1 | |
Record name | 4-chlorobenzothiazole | |
Source | EPA DSSTox | |
URL | https://comptox.epa.gov/dashboard/DTXSID70445303 | |
Description | DSSTox provides a high quality public chemistry resource for supporting improved predictive toxicology. | |
Retrosynthesis Analysis
AI-Powered Synthesis Planning: Our tool employs the Template_relevance Pistachio, Template_relevance Bkms_metabolic, Template_relevance Pistachio_ringbreaker, Template_relevance Reaxys, Template_relevance Reaxys_biocatalysis model, leveraging a vast database of chemical reactions to predict feasible synthetic routes.
One-Step Synthesis Focus: Specifically designed for one-step synthesis, it provides concise and direct routes for your target compounds, streamlining the synthesis process.
Accurate Predictions: Utilizing the extensive PISTACHIO, BKMS_METABOLIC, PISTACHIO_RINGBREAKER, REAXYS, REAXYS_BIOCATALYSIS database, our tool offers high-accuracy predictions, reflecting the latest in chemical research and data.
Strategy Settings
Precursor scoring | Relevance Heuristic |
---|---|
Min. plausibility | 0.01 |
Model | Template_relevance |
Template Set | Pistachio/Bkms_metabolic/Pistachio_ringbreaker/Reaxys/Reaxys_biocatalysis |
Top-N result to add to graph | 6 |
Feasible Synthetic Routes
Q1: What are the potential applications of 4-chlorobenzothiazole derivatives in material science?
A1: Research indicates that 2-amino-4-chlorobenzothiazole (ACBT) demonstrates promising corrosion inhibition properties for X65 steel in sulfuric acid (H2SO4) solutions. [] Electrochemical studies reveal that ACBT acts as a mixed-type corrosion inhibitor, effectively suppressing both anodic and cathodic reactions on the steel surface. [] At a concentration of 5 mM, ACBT achieves a corrosion inhibition efficiency close to 90% at 298 K. [] This protective effect is attributed to the adsorption of ACBT onto the steel surface, forming a barrier against corrosive agents. [] Surface morphology analysis using Scanning Electron Microscopy (SEM) supports these findings, showing significantly reduced corrosion on steel surfaces treated with ACBT. []
Q2: How does the structure of this compound-derived Schiff bases contribute to their biological activity?
A2: Schiff bases derived from this compound exhibit promising antibacterial activity. [] These compounds, characterized by the presence of an azomethine linkage (CH=N), readily form complexes with metal ions like Zn(II). [] This coordination is believed to play a crucial role in their biological activity, potentially by interfering with essential metal-dependent processes in bacteria. [] Studies have shown that these Schiff base-metal complexes exhibit antibacterial activity against Escherichia coli, Staphylococcus aureus, and Pseudomonas aeruginosa. []
Q3: What insights can computational chemistry provide into the properties of this compound derivatives?
A3: Computational methods, including quantum chemical calculations and molecular dynamics simulations, have proven valuable in understanding the corrosion inhibition mechanism of ACBT. [] These simulations can elucidate the interaction energies and adsorption behavior of ACBT on metal surfaces, providing insights into its effectiveness as a corrosion inhibitor. [] Such computational approaches complement experimental findings and guide the development of new and improved corrosion inhibitors. []
Q4: What is the significance of the Langmuir adsorption isothermal model in the context of this compound's corrosion inhibition?
A4: The adsorption of ACBT onto the X65 steel surface follows the Langmuir adsorption isothermal model. [] This suggests that ACBT molecules adsorb onto specific active sites on the steel surface, forming a monolayer. [] Understanding the adsorption behavior is crucial for optimizing the concentration and effectiveness of ACBT as a corrosion inhibitor in various applications. []
Avertissement et informations sur les produits de recherche in vitro
Veuillez noter que tous les articles et informations sur les produits présentés sur BenchChem sont destinés uniquement à des fins informatives. Les produits disponibles à l'achat sur BenchChem sont spécifiquement conçus pour des études in vitro, qui sont réalisées en dehors des organismes vivants. Les études in vitro, dérivées du terme latin "in verre", impliquent des expériences réalisées dans des environnements de laboratoire contrôlés à l'aide de cellules ou de tissus. Il est important de noter que ces produits ne sont pas classés comme médicaments et n'ont pas reçu l'approbation de la FDA pour la prévention, le traitement ou la guérison de toute condition médicale, affection ou maladie. Nous devons souligner que toute forme d'introduction corporelle de ces produits chez les humains ou les animaux est strictement interdite par la loi. Il est essentiel de respecter ces directives pour assurer la conformité aux normes légales et éthiques en matière de recherche et d'expérimentation.