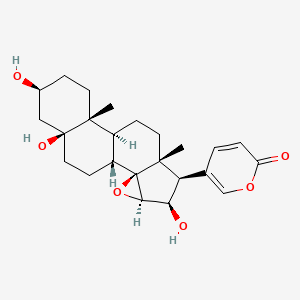
Désacétylcinoboufootaline
Vue d'ensemble
Description
La désacétylcinobufotaline est un composé naturel dérivé de la peau du crapaud Bufo bufo gargarizans Cantor. Elle appartient à la classe des bufadiénolides, connus pour leurs activités biologiques puissantes, y compris les propriétés anticancéreuses . Ce composé a été étudié pour sa capacité à induire l'apoptose dans les cellules cancéreuses, ce qui en fait un sujet d'intérêt pour la recherche pharmacologique .
Applications De Recherche Scientifique
La désacétylcinobufotaline a une large gamme d'applications en recherche scientifique :
Chimie : Elle est utilisée comme composé modèle pour étudier la réactivité et la stabilité des bufadiénolides.
Mécanisme d'action
La désacétylcinobufotaline exerce ses effets principalement par l'induction de l'apoptose dans les cellules cancéreuses. Elle cible des voies moléculaires spécifiques impliquées dans la mort cellulaire, y compris l'activation des caspases et la perturbation de la fonction mitochondriale . Le composé se lie et inhibe des protéines clés impliquées dans la survie cellulaire, ce qui conduit à la mort cellulaire programmée .
Orientations Futures
The future of synthetic chemistry, which includes the synthesis of compounds like De-O-acetylcinobufotalin, is expected to focus on higher selectivity, higher efficiency, environmental benignity, and sustainable energy . The discovery of high-performance catalysts will evolve from trial-and-error to rational design .
Mécanisme D'action
Target of Action
Desacetylcinobufotalin, also known as De-O-acetylcinobufotalin, is a natural compound that has been found to have significant antitumor activity . The primary targets of Desacetylcinobufotalin are cancer cells, particularly HepG2 cells . It induces apoptosis in these cells, leading to their death .
Mode of Action
Desacetylcinobufotalin interacts with its targets by inducing apoptosis via the mitochondria pathway . This interaction results in significant changes within the target cells, including the marked inhibition of HepG2 cells . The IC50 value, a measure of the effectiveness of Desacetylcinobufotalin in inhibiting biological function, is 0.0279μmol/ml for HepG2 cells .
Biochemical Pathways
Desacetylcinobufotalin affects several biochemical pathways. It has been found to dysregulate cell proliferation, which is a key mechanism in its anti-tumor effects . Network pharmacology analyses suggest that Desacetylcinobufotalin may inhibit hepatocellular carcinoma (HCC) via directly modulating cancer, metabolism, and immune-related pathways .
Pharmacokinetics
The pharmacokinetics of Desacetylcinobufotalin, including its Absorption, Distribution, Metabolism, and Excretion (ADME) properties, impact its bioavailability . The pharmacokinetic characteristics of Desacetylcinobufotalin vary widely, which could explain differences in the efficacy of different formulations .
Result of Action
The molecular and cellular effects of Desacetylcinobufotalin’s action are significant. It has been found to induce apoptosis in HepG2 cells, leading to their death . This results in a marked inhibition effect on these cells .
Analyse Biochimique
Biochemical Properties
Desacetylcinobufotalin plays a crucial role in biochemical reactions, primarily as an apoptosis inducer. It interacts with various enzymes, proteins, and other biomolecules to exert its effects. One of the key interactions is with the mitochondrial pathway, where desacetylcinobufotalin increases the expression of pro-apoptotic proteins such as Bax and decreases the expression of anti-apoptotic proteins like Bcl-2 . This interaction leads to the activation of caspases, which are essential for the execution of apoptosis. Additionally, desacetylcinobufotalin has been shown to inhibit the activity of certain kinases involved in cell survival pathways, further promoting apoptosis in cancer cells .
Cellular Effects
Desacetylcinobufotalin exerts significant effects on various types of cells and cellular processes. In cancer cells, it induces apoptosis by disrupting the mitochondrial membrane potential and activating the caspase cascade . This compound also influences cell signaling pathways, such as the PI3K-AKT and MAPK pathways, which are critical for cell proliferation and survival . By inhibiting these pathways, desacetylcinobufotalin reduces cell proliferation and promotes cell death. Furthermore, it affects gene expression by modulating the transcription of genes involved in apoptosis and cell cycle regulation . In addition to its anti-cancer effects, desacetylcinobufotalin has been reported to impact cellular metabolism by altering the levels of key metabolites and enzymes involved in metabolic pathways .
Molecular Mechanism
The molecular mechanism of desacetylcinobufotalin involves several key steps. At the molecular level, desacetylcinobufotalin binds to and inhibits the activity of specific kinases, such as PI3K and AKT, which are involved in cell survival and proliferation pathways . This inhibition leads to the activation of downstream effectors, including the pro-apoptotic proteins Bax and caspases, resulting in apoptosis . Additionally, desacetylcinobufotalin modulates the expression of genes involved in apoptosis and cell cycle regulation by interacting with transcription factors and other regulatory proteins . These molecular interactions collectively contribute to the anti-cancer effects of desacetylcinobufotalin.
Temporal Effects in Laboratory Settings
In laboratory settings, the effects of desacetylcinobufotalin have been observed to change over time. The stability and degradation of desacetylcinobufotalin are critical factors that influence its long-term effects on cellular function. Studies have shown that desacetylcinobufotalin remains stable under specific storage conditions, such as -20°C for up to one month and -80°C for up to six months . Over time, desacetylcinobufotalin continues to induce apoptosis in cancer cells, with sustained inhibition of cell proliferation and survival pathways . Long-term studies in vitro and in vivo have demonstrated that desacetylcinobufotalin maintains its anti-cancer activity, although the extent of its effects may vary depending on the experimental conditions and duration of treatment .
Dosage Effects in Animal Models
The effects of desacetylcinobufotalin vary with different dosages in animal models. At lower doses, desacetylcinobufotalin has been shown to induce apoptosis and inhibit tumor growth without causing significant toxicity . At higher doses, desacetylcinobufotalin may exhibit toxic effects, including cardiotoxicity and hepatotoxicity . Threshold effects have been observed, where a certain dosage is required to achieve the desired therapeutic effects, while exceeding this threshold may lead to adverse effects. Therefore, careful dosage optimization is essential to maximize the therapeutic benefits of desacetylcinobufotalin while minimizing its toxicity in animal models.
Metabolic Pathways
Desacetylcinobufotalin is involved in several metabolic pathways, interacting with various enzymes and cofactors. It has been reported to affect the metabolism of key metabolites, such as ATP and NADH, by modulating the activity of enzymes involved in energy production and oxidative phosphorylation . Additionally, desacetylcinobufotalin influences the levels of reactive oxygen species (ROS) and antioxidant enzymes, contributing to its pro-apoptotic effects . The metabolic pathways affected by desacetylcinobufotalin are critical for its anti-cancer activity, as they regulate cellular energy balance and redox homeostasis.
Transport and Distribution
Desacetylcinobufotalin is transported and distributed within cells and tissues through specific transporters and binding proteins. It has been shown to interact with efflux transporters, such as P-glycoprotein, which play a role in its cellular uptake and distribution . The localization and accumulation of desacetylcinobufotalin within cells are influenced by these transporters, affecting its bioavailability and therapeutic efficacy . Additionally, desacetylcinobufotalin may bind to plasma proteins, which can impact its distribution and clearance from the body .
Subcellular Localization
The subcellular localization of desacetylcinobufotalin is crucial for its activity and function. Desacetylcinobufotalin has been reported to localize primarily in the mitochondria, where it exerts its pro-apoptotic effects by disrupting the mitochondrial membrane potential and activating the caspase cascade . Targeting signals and post-translational modifications may direct desacetylcinobufotalin to specific cellular compartments, enhancing its therapeutic efficacy . Understanding the subcellular localization of desacetylcinobufotalin is essential for elucidating its mechanism of action and optimizing its use in cancer therapy.
Méthodes De Préparation
Voies de synthèse et conditions réactionnelles
La désacétylcinobufotaline peut être synthétisée par hydrolyse de la cinobufotaline, qui implique l'élimination d'un groupe acétyle. La réaction nécessite généralement des conditions acides ou basiques pour faciliter le processus d'hydrolyse . La voie de synthèse implique l'utilisation de solvants tels que le méthanol ou l'éthanol, et la réaction est souvent effectuée à des températures élevées pour assurer une conversion complète .
Méthodes de production industrielle
La production industrielle de désacétylcinobufotaline implique l'extraction de la cinobufotaline de la peau de Bufo bufo gargarizans Cantor, suivie d'une hydrolyse pour obtenir la désacétylcinobufotaline . Le processus d'extraction comprend des étapes telles que le séchage, le broyage et l'extraction par solvant, suivies d'une purification à l'aide de techniques chromatographiques .
Analyse Des Réactions Chimiques
Types de réactions
La désacétylcinobufotaline subit diverses réactions chimiques, notamment :
Oxydation : Elle peut être oxydée pour former des intermédiaires plus réactifs.
Réduction : Les réactions de réduction peuvent la convertir en formes moins oxydées.
Substitution : Elle peut subir des réactions de substitution où des groupes fonctionnels sont remplacés par d'autres groupes.
Réactifs et conditions courants
Oxydation : Les agents oxydants courants comprennent le permanganate de potassium et le peroxyde d'hydrogène.
Réduction : Des agents réducteurs tels que le borohydrure de sodium et l'hydrure de lithium et d'aluminium sont utilisés.
Substitution : Des réactifs tels que les halogènes et les nucléophiles sont utilisés dans les réactions de substitution.
Principaux produits formés
Les principaux produits formés à partir de ces réactions dépendent des conditions et des réactifs spécifiques utilisés. Par exemple, l'oxydation peut conduire à la formation d'intermédiaires plus réactifs, tandis que la réduction peut produire des formes moins oxydées de désacétylcinobufotaline .
Comparaison Avec Des Composés Similaires
Composés similaires
Cinobufotaline : Le composé parent dont la désacétylcinobufotaline est dérivée.
Bufotaline : Un autre bufadiénolide possédant des propriétés anticancéreuses similaires.
Résibufogénine : Un composé apparenté ayant des activités biologiques puissantes.
Unicité
La désacétylcinobufotaline est unique en raison de sa structure spécifique, qui ne possède pas de groupe acétyle par rapport à la cinobufotaline. Cette différence structurelle contribue à ses activités biologiques et propriétés pharmacocinétiques distinctes . Sa capacité à induire l'apoptose dans les cellules cancéreuses avec une grande puissance la distingue des autres composés similaires .
Propriétés
Numéro CAS |
4099-30-3 |
---|---|
Formule moléculaire |
C24H32O6 |
Poids moléculaire |
416.5 g/mol |
Nom IUPAC |
5-[(2S,4R,5R,6R,7R,11R,14S,16S)-5,14,16-trihydroxy-7,11-dimethyl-3-oxapentacyclo[8.8.0.02,4.02,7.011,16]octadecan-6-yl]pyran-2-one |
InChI |
InChI=1S/C24H32O6/c1-21-8-5-14(25)11-23(21,28)10-7-16-15(21)6-9-22(2)18(13-3-4-17(26)29-12-13)19(27)20-24(16,22)30-20/h3-4,12,14-16,18-20,25,27-28H,5-11H2,1-2H3/t14-,15?,16?,18-,19+,20+,21+,22+,23-,24+/m0/s1 |
Clé InChI |
FRYICJTUIXEEGK-VGKSGQJVSA-N |
SMILES isomérique |
C[C@]12CCC3C([C@@]14[C@H](O4)[C@@H]([C@@H]2C5=COC(=O)C=C5)O)CC[C@]6([C@@]3(CC[C@@H](C6)O)C)O |
SMILES |
CC12CCC(CC1(CCC3C2CCC4(C35C(O5)C(C4C6=COC(=O)C=C6)O)C)O)O |
SMILES canonique |
CC12CCC(CC1(CCC3C2CCC4(C35C(O5)C(C4C6=COC(=O)C=C6)O)C)O)O |
Origine du produit |
United States |
Retrosynthesis Analysis
AI-Powered Synthesis Planning: Our tool employs the Template_relevance Pistachio, Template_relevance Bkms_metabolic, Template_relevance Pistachio_ringbreaker, Template_relevance Reaxys, Template_relevance Reaxys_biocatalysis model, leveraging a vast database of chemical reactions to predict feasible synthetic routes.
One-Step Synthesis Focus: Specifically designed for one-step synthesis, it provides concise and direct routes for your target compounds, streamlining the synthesis process.
Accurate Predictions: Utilizing the extensive PISTACHIO, BKMS_METABOLIC, PISTACHIO_RINGBREAKER, REAXYS, REAXYS_BIOCATALYSIS database, our tool offers high-accuracy predictions, reflecting the latest in chemical research and data.
Strategy Settings
Precursor scoring | Relevance Heuristic |
---|---|
Min. plausibility | 0.01 |
Model | Template_relevance |
Template Set | Pistachio/Bkms_metabolic/Pistachio_ringbreaker/Reaxys/Reaxys_biocatalysis |
Top-N result to add to graph | 6 |
Feasible Synthetic Routes
Avertissement et informations sur les produits de recherche in vitro
Veuillez noter que tous les articles et informations sur les produits présentés sur BenchChem sont destinés uniquement à des fins informatives. Les produits disponibles à l'achat sur BenchChem sont spécifiquement conçus pour des études in vitro, qui sont réalisées en dehors des organismes vivants. Les études in vitro, dérivées du terme latin "in verre", impliquent des expériences réalisées dans des environnements de laboratoire contrôlés à l'aide de cellules ou de tissus. Il est important de noter que ces produits ne sont pas classés comme médicaments et n'ont pas reçu l'approbation de la FDA pour la prévention, le traitement ou la guérison de toute condition médicale, affection ou maladie. Nous devons souligner que toute forme d'introduction corporelle de ces produits chez les humains ou les animaux est strictement interdite par la loi. Il est essentiel de respecter ces directives pour assurer la conformité aux normes légales et éthiques en matière de recherche et d'expérimentation.