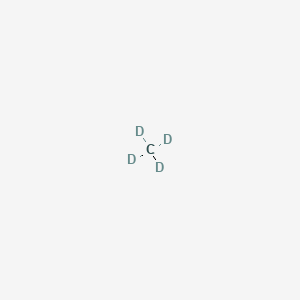
Methane-d4
Vue d'ensemble
Description
Methane-d4, also known as deuterated methane, is a compound with the chemical formula CD4. It is a form of methane where all four hydrogen atoms are replaced by deuterium, a stable isotope of hydrogen. This compound is of significant interest in various scientific fields due to its unique properties and applications.
Méthodes De Préparation
Synthetic Routes and Reaction Conditions
Methane-d4 can be synthesized through several methods. One common approach involves the reaction of deuterium gas (D2) with carbon at high temperatures. Another method includes the exchange reaction between methane (CH4) and heavy water (D2O) in the presence of a catalyst. The reaction conditions typically involve elevated temperatures and pressures to facilitate the exchange of hydrogen atoms with deuterium.
Industrial Production Methods
Industrial production of this compound often involves the catalytic exchange process, where methane is reacted with deuterium oxide in the presence of a catalyst such as platinum or palladium. This method is efficient and allows for the large-scale production of deuterated methane.
Analyse Des Réactions Chimiques
Types of Reactions
Methane-d4 undergoes various chemical reactions, including:
Oxidation: When this compound is oxidized, it forms deuterated carbon dioxide (C(D2)O2) and heavy water (D2O).
Substitution: In substitution reactions, one or more deuterium atoms in this compound can be replaced by other atoms or groups, such as halogens.
Combustion: this compound combusts in the presence of oxygen to produce deuterated water and carbon dioxide.
Common Reagents and Conditions
Oxidation: Requires an oxidizing agent such as oxygen or ozone.
Substitution: Typically involves halogens like chlorine or bromine in the presence of light or heat.
Combustion: Requires oxygen and an ignition source.
Major Products
Oxidation: Deuterated carbon dioxide and heavy water.
Substitution: Deuterated halomethanes.
Combustion: Deuterated water and carbon dioxide.
Applications De Recherche Scientifique
Methane-d4 has numerous applications in scientific research:
Chemistry: Used as a tracer in reaction mechanisms to study the behavior of deuterium in chemical reactions.
Biology: Employed in metabolic studies to trace the incorporation of deuterium into biological molecules.
Medicine: Utilized in magnetic resonance imaging (MRI) as a contrast agent due to its unique magnetic properties.
Industry: Applied in the production of deuterated compounds, which are used in various industrial processes.
Mécanisme D'action
The mechanism by which Methane-d4 exerts its effects is primarily through the replacement of hydrogen atoms with deuterium. This substitution alters the physical and chemical properties of the compound, such as its bond strength and reaction rates. The presence of deuterium can slow down reaction rates due to the kinetic isotope effect, making this compound a valuable tool in studying reaction mechanisms and pathways.
Comparaison Avec Des Composés Similaires
Similar Compounds
Methane (CH4): The non-deuterated form of Methane-d4.
Deuterated Ethane (C2D6): Another deuterated hydrocarbon with similar applications.
Deuterated Propane (C3D8): A deuterated form of propane used in similar research applications.
Uniqueness
This compound is unique due to its complete substitution of hydrogen with deuterium, which significantly alters its physical and chemical properties. This makes it particularly useful in studies requiring precise control over isotopic composition and reaction kinetics.
Propriétés
IUPAC Name |
tetradeuteriomethane | |
---|---|---|
Source | PubChem | |
URL | https://pubchem.ncbi.nlm.nih.gov | |
Description | Data deposited in or computed by PubChem | |
InChI |
InChI=1S/CH4/h1H4/i1D4 | |
Source | PubChem | |
URL | https://pubchem.ncbi.nlm.nih.gov | |
Description | Data deposited in or computed by PubChem | |
InChI Key |
VNWKTOKETHGBQD-JQYAHLJZSA-N | |
Source | PubChem | |
URL | https://pubchem.ncbi.nlm.nih.gov | |
Description | Data deposited in or computed by PubChem | |
Canonical SMILES |
C | |
Source | PubChem | |
URL | https://pubchem.ncbi.nlm.nih.gov | |
Description | Data deposited in or computed by PubChem | |
Isomeric SMILES |
[2H]C([2H])([2H])[2H] | |
Source | PubChem | |
URL | https://pubchem.ncbi.nlm.nih.gov | |
Description | Data deposited in or computed by PubChem | |
Molecular Formula |
CH4 | |
Source | PubChem | |
URL | https://pubchem.ncbi.nlm.nih.gov | |
Description | Data deposited in or computed by PubChem | |
DSSTOX Substance ID |
DTXSID30204377 | |
Record name | (2H4)Methane | |
Source | EPA DSSTox | |
URL | https://comptox.epa.gov/dashboard/DTXSID30204377 | |
Description | DSSTox provides a high quality public chemistry resource for supporting improved predictive toxicology. | |
Molecular Weight |
20.067 g/mol | |
Source | PubChem | |
URL | https://pubchem.ncbi.nlm.nih.gov | |
Description | Data deposited in or computed by PubChem | |
Physical Description |
Compressed gas; [Sigma-Aldrich MSDS] | |
Record name | Methane-d4 | |
Source | Haz-Map, Information on Hazardous Chemicals and Occupational Diseases | |
URL | https://haz-map.com/Agents/13659 | |
Description | Haz-Map® is an occupational health database designed for health and safety professionals and for consumers seeking information about the adverse effects of workplace exposures to chemical and biological agents. | |
Explanation | Copyright (c) 2022 Haz-Map(R). All rights reserved. Unless otherwise indicated, all materials from Haz-Map are copyrighted by Haz-Map(R). No part of these materials, either text or image may be used for any purpose other than for personal use. Therefore, reproduction, modification, storage in a retrieval system or retransmission, in any form or by any means, electronic, mechanical or otherwise, for reasons other than personal use, is strictly prohibited without prior written permission. | |
CAS No. |
558-20-3 | |
Record name | Methane-d4 | |
Source | CAS Common Chemistry | |
URL | https://commonchemistry.cas.org/detail?cas_rn=558-20-3 | |
Description | CAS Common Chemistry is an open community resource for accessing chemical information. Nearly 500,000 chemical substances from CAS REGISTRY cover areas of community interest, including common and frequently regulated chemicals, and those relevant to high school and undergraduate chemistry classes. This chemical information, curated by our expert scientists, is provided in alignment with our mission as a division of the American Chemical Society. | |
Explanation | The data from CAS Common Chemistry is provided under a CC-BY-NC 4.0 license, unless otherwise stated. | |
Record name | (2H4)Methane | |
Source | ChemIDplus | |
URL | https://pubchem.ncbi.nlm.nih.gov/substance/?source=chemidplus&sourceid=0000558203 | |
Description | ChemIDplus is a free, web search system that provides access to the structure and nomenclature authority files used for the identification of chemical substances cited in National Library of Medicine (NLM) databases, including the TOXNET system. | |
Record name | (2H4)Methane | |
Source | EPA DSSTox | |
URL | https://comptox.epa.gov/dashboard/DTXSID30204377 | |
Description | DSSTox provides a high quality public chemistry resource for supporting improved predictive toxicology. | |
Record name | (2H4)methane | |
Source | European Chemicals Agency (ECHA) | |
URL | https://echa.europa.eu/substance-information/-/substanceinfo/100.008.357 | |
Description | The European Chemicals Agency (ECHA) is an agency of the European Union which is the driving force among regulatory authorities in implementing the EU's groundbreaking chemicals legislation for the benefit of human health and the environment as well as for innovation and competitiveness. | |
Explanation | Use of the information, documents and data from the ECHA website is subject to the terms and conditions of this Legal Notice, and subject to other binding limitations provided for under applicable law, the information, documents and data made available on the ECHA website may be reproduced, distributed and/or used, totally or in part, for non-commercial purposes provided that ECHA is acknowledged as the source: "Source: European Chemicals Agency, http://echa.europa.eu/". Such acknowledgement must be included in each copy of the material. ECHA permits and encourages organisations and individuals to create links to the ECHA website under the following cumulative conditions: Links can only be made to webpages that provide a link to the Legal Notice page. | |
Synthesis routes and methods I
Procedure details
Synthesis routes and methods II
Procedure details
Synthesis routes and methods III
Procedure details
Synthesis routes and methods IV
Procedure details
Synthesis routes and methods V
Procedure details
Retrosynthesis Analysis
AI-Powered Synthesis Planning: Our tool employs the Template_relevance Pistachio, Template_relevance Bkms_metabolic, Template_relevance Pistachio_ringbreaker, Template_relevance Reaxys, Template_relevance Reaxys_biocatalysis model, leveraging a vast database of chemical reactions to predict feasible synthetic routes.
One-Step Synthesis Focus: Specifically designed for one-step synthesis, it provides concise and direct routes for your target compounds, streamlining the synthesis process.
Accurate Predictions: Utilizing the extensive PISTACHIO, BKMS_METABOLIC, PISTACHIO_RINGBREAKER, REAXYS, REAXYS_BIOCATALYSIS database, our tool offers high-accuracy predictions, reflecting the latest in chemical research and data.
Strategy Settings
Precursor scoring | Relevance Heuristic |
---|---|
Min. plausibility | 0.01 |
Model | Template_relevance |
Template Set | Pistachio/Bkms_metabolic/Pistachio_ringbreaker/Reaxys/Reaxys_biocatalysis |
Top-N result to add to graph | 6 |
Feasible Synthetic Routes
ANone: Methane-d4 has the molecular formula CD4 and a molecular weight of 20.03 g/mol.
A: The substitution of deuterium for hydrogen in this compound leads to significant changes in its spectroscopic properties. [, , , ] These differences arise from the mass difference between the isotopes, affecting vibrational frequencies and rotational constants. For instance, the Raman spectrum of CD4 exhibits distinct rotational structures in the ν2 and ν3 bands compared to CH4. [] Additionally, electron impact spectroscopy reveals variations in the bound state spectrum and optically forbidden vibrational progressions between the two isotopologues. [, ]
A: Research suggests that this compound undergoes dissociative adsorption on nickel catalysts. [] Kinetic studies indicate a first-order disappearance of this compound on the nickel surface, with the formation of surface-bound fragments like CX, CX2, and CX3 (where X is H or D). [] The equilibrium between these fragments is influenced by temperature, with higher temperatures favoring CX3. []
A: this compound is a valuable probe for studying H/D exchange reactions, particularly in zeolites and other catalytic systems. [, ] By monitoring the exchange of deuterium from this compound with hydrogen atoms on Brønsted acid sites using techniques like solid-state NMR, researchers can gain insights into the kinetics and mechanisms of these reactions. [, ] For example, studies using this compound have revealed that InO+/H-ZSM-5 zeolites exhibit significantly faster H/D exchange rates compared to In+/H-ZSM-5 or pure H-ZSM-5 zeolites. []
A: Neutron diffraction studies reveal that this compound forms an ordered two-dimensional layer on graphitized carbon black surfaces, specifically Vulcan III. [] This layer aligns in a √3 × √3 registry with the graphite basal planes. [] The study also provided insights into the methane-surface distance and the influence of coverage and temperature on layer formation. []
ANone: The provided research papers do not delve into detailed material compatibility studies for this compound.
A: Yes, DFT (Density Functional Theory) calculations have been employed to study the interaction of platinum ions (Pt+) with this compound. [] These calculations provided evidence for the formation of a platinum hydrido-methyl complex (HPt+CH3) during these reactions. []
ANone: While isotopic substitution doesn't inherently make a molecule more toxic, it's always crucial to handle all chemicals with care. As this compound is a gas, ensure proper ventilation and avoid inhalation. Consult the material safety data sheet (MSDS) for comprehensive safety information.
ANone: Various analytical methods are valuable for studying this compound and its interactions. Some key techniques highlighted in the research include:
- Mass Spectrometry: Used to identify and quantify this compound and its reaction products based on their mass-to-charge ratios. [, , , , ]
- Nuclear Magnetic Resonance (NMR) Spectroscopy: Particularly solid-state NMR, enables the study of H/D exchange reactions involving this compound and provides insights into molecular dynamics and interactions. [, ]
- Neutron Diffraction: Provides structural information, especially for adsorbed species on surfaces, as demonstrated by the study of this compound on graphitized carbon black. []
- Electron Impact Spectroscopy: Used to investigate electronic structures and vibrational modes of this compound. [, ]
- Infrared Spectroscopy: Valuable for studying molecular vibrations and interactions, as seen in studies of this compound van der Waals clusters. []
A: Early studies on this compound primarily focused on its spectroscopic properties and fundamental molecular characteristics. [, , , ] As analytical techniques advanced, the research expanded to explore its interactions with catalytic surfaces, [, , , , ] investigate reaction mechanisms, [, , , , ] and probe the dynamics of H/D exchange processes. [, ]
Avertissement et informations sur les produits de recherche in vitro
Veuillez noter que tous les articles et informations sur les produits présentés sur BenchChem sont destinés uniquement à des fins informatives. Les produits disponibles à l'achat sur BenchChem sont spécifiquement conçus pour des études in vitro, qui sont réalisées en dehors des organismes vivants. Les études in vitro, dérivées du terme latin "in verre", impliquent des expériences réalisées dans des environnements de laboratoire contrôlés à l'aide de cellules ou de tissus. Il est important de noter que ces produits ne sont pas classés comme médicaments et n'ont pas reçu l'approbation de la FDA pour la prévention, le traitement ou la guérison de toute condition médicale, affection ou maladie. Nous devons souligner que toute forme d'introduction corporelle de ces produits chez les humains ou les animaux est strictement interdite par la loi. Il est essentiel de respecter ces directives pour assurer la conformité aux normes légales et éthiques en matière de recherche et d'expérimentation.