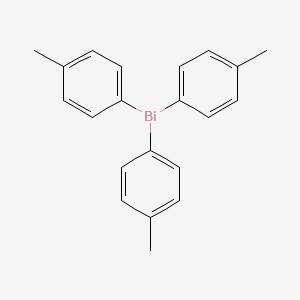
Tri-p-tolyl bismuthine
Vue d'ensemble
Description
It is characterized by its white crystalline solid appearance and has a melting point of 119°C . This compound is known for its applications in organic synthesis, particularly as a catalyst.
Applications De Recherche Scientifique
Organic Synthesis
Tri-p-tolylbismuthine is utilized as a reagent in organic synthesis. Its distinctive structure allows it to facilitate various chemical reactions, particularly those involving electrophiles. It serves as a source of bismuth, which can stabilize intermediates and promote reaction pathways that may not be accessible with other reagents.
Key Reactions:
- Bismuth-Catalyzed Reactions: The compound is effective in promoting reactions such as the formation of carbon-carbon bonds and the synthesis of complex organic molecules. Its ability to stabilize radical intermediates makes it valuable in radical chemistry.
- Electrophilic Aromatic Substitution: Tri-p-tolylbismuthine can act as a catalyst in electrophilic aromatic substitution reactions, enhancing the reactivity of aromatic compounds.
Pharmaceutical Applications
The pharmaceutical industry has shown interest in tri-p-tolylbismuthine due to its potential as a precursor for drug development. Bismuth compounds are known for their antimicrobial properties, and tri-p-tolylbismuthine can be modified to create derivatives that may exhibit enhanced biological activity.
Case Studies:
- Antibacterial Agents: Research has indicated that bismuth compounds can possess antibacterial properties. Tri-p-tolylbismuthine derivatives have been studied for their efficacy against various bacterial strains.
- Cancer Research: There is emerging evidence that bismuth-based compounds may play a role in cancer therapy, potentially acting as chemotherapeutic agents or enhancing the effectiveness of existing treatments.
Materials Science
In materials science, tri-p-tolylbismuthine is being explored for its applications in thin film deposition and electronic materials. Its organometallic nature allows for the formation of thin films that can be used in various electronic devices.
Applications:
- Thin Film Deposition: The compound can be used in the deposition of bismuth-containing thin films, which are essential for applications in semiconductors and optoelectronics.
- LED Technology: Tri-p-tolylbismuthine has potential applications in the development of light-emitting diodes (LEDs) due to its favorable electronic properties.
Summary Table of Applications
Application Area | Description | Examples/Case Studies |
---|---|---|
Organic Synthesis | Reagent for carbon-carbon bond formation and electrophilic substitutions | Bismuth-catalyzed reactions |
Pharmaceuticals | Potential precursor for antibacterial and anticancer drugs | Antibacterial studies |
Materials Science | Used in thin film deposition for electronic devices | Development of semiconductors and LEDs |
Mécanisme D'action
Target of Action
Tri-p-tolylbismuthine, also known as Tris(4-methylphenyl)bismuthine or tris(4-methylphenyl)bismuthane, is primarily targeted towards the development of superconductors . The compound’s primary targets are the potassium ions and the carbon atoms in the methylphenyl groups .
Mode of Action
The mode of action of Tri-p-tolylbismuthine involves the transfer of an electron from the potassium ions (K 4s) to the carbon atoms in the methylphenyl groups (C 2p orbital) . This electron transfer results in both red and blue shifts of the Raman spectra .
Biochemical Pathways
The biochemical pathway affected by Tri-p-tolylbismuthine is the superconductivity pathway . The compound, when doped with potassium, exhibits type-II superconductivity at 3.6 K at ambient pressure . This superconductivity is a result of the electron transfer from the potassium ions to the carbon atoms in the methylphenyl groups .
Result of Action
The result of Tri-p-tolylbismuthine’s action is the exhibition of type-II superconductivity at 3.6 K at ambient pressure . This superconductivity is due to the electron transfer from the potassium ions to the carbon atoms in the methylphenyl groups .
Action Environment
The action of Tri-p-tolylbismuthine is influenced by environmental factors such as temperature and pressure . The compound exhibits type-II superconductivity at 3.6 K at ambient pressure . Therefore, changes in these environmental factors could potentially influence the compound’s action, efficacy, and stability.
Analyse Biochimique
Biochemical Properties
Tris(4-methylphenyl)bismuthine plays a significant role in biochemical reactions, particularly as a catalyst. It interacts with various enzymes and proteins, influencing their activity. For instance, it has been observed to affect the stability of immobilized enzymes such as trypsin, chymotrypsin, and penicillin G acylase when used in the presence of Tris buffer . The interactions between Tris(4-methylphenyl)bismuthine and these enzymes can lead to a reduction in enzyme stability, which is attributed to steric hindrances and the reduction of available glyoxyl groups during immobilization .
Cellular Effects
Tris(4-methylphenyl)bismuthine has notable effects on various cell types and cellular processes. It has been shown to induce cytotoxicity in cancer cell lines by inhibiting human glyoxalase I, leading to the accumulation of methylglyoxal and subsequent cell death . This compound also influences cell signaling pathways, gene expression, and cellular metabolism, making it a potential candidate for cancer therapy .
Molecular Mechanism
The molecular mechanism of action of Tris(4-methylphenyl)bismuthine involves its interaction with biomolecules, leading to enzyme inhibition and changes in gene expression. It binds to human glyoxalase I, inhibiting its activity and causing the accumulation of methylglyoxal, which induces cytotoxic effects in cancer cells . Additionally, Tris(4-methylphenyl)bismuthine can act as a catalyst in organic synthesis, facilitating reactions such as the hydrogenation of benzophenone .
Temporal Effects in Laboratory Settings
In laboratory settings, the effects of Tris(4-methylphenyl)bismuthine can change over time. Its stability and degradation are crucial factors that influence its long-term effects on cellular function. Studies have shown that the stability of immobilized enzymes decreases in the presence of Tris buffer, with the reduction in enzyme stability being more pronounced at higher concentrations of Tris . This suggests that the temporal effects of Tris(4-methylphenyl)bismuthine are influenced by its interaction with other compounds and environmental conditions.
Dosage Effects in Animal Models
The effects of Tris(4-methylphenyl)bismuthine vary with different dosages in animal models. At lower doses, it may exhibit beneficial effects, such as enzyme inhibition and cytotoxicity in cancer cells. At higher doses, it can cause toxic or adverse effects, including potential toxicity to normal cells and tissues . Understanding the dosage effects is essential for determining the therapeutic window and safety profile of Tris(4-methylphenyl)bismuthine.
Metabolic Pathways
Tris(4-methylphenyl)bismuthine is involved in various metabolic pathways, interacting with enzymes and cofactors that influence metabolic flux and metabolite levels. For example, it has been shown to interact with glyoxalase I, leading to the accumulation of methylglyoxal and affecting cellular metabolism . The degradation pathway of Tris buffer in bacteria also highlights the potential metabolic interactions of Tris(4-methylphenyl)bismuthine .
Transport and Distribution
The transport and distribution of Tris(4-methylphenyl)bismuthine within cells and tissues are influenced by its interactions with transporters and binding proteins. Studies have shown that it can be transported and localized within specific cellular compartments, affecting its accumulation and activity . Understanding the transport mechanisms is crucial for determining the bioavailability and efficacy of Tris(4-methylphenyl)bismuthine.
Subcellular Localization
Tris(4-methylphenyl)bismuthine exhibits specific subcellular localization, which can influence its activity and function. It may be directed to particular compartments or organelles through targeting signals or post-translational modifications . The subcellular localization of Tris(4-methylphenyl)bismuthine is essential for understanding its mechanism of action and potential therapeutic applications.
Méthodes De Préparation
Tri-p-tolylbismuthine can be synthesized through various methods. One common synthetic route involves the reaction of bismuth trichloride with 4-methylphenylmagnesium bromide in the presence of a catalyst . The reaction conditions typically include an inert atmosphere and a solvent such as tetrahydrofuran (THF). Industrial production methods may involve similar reactions but on a larger scale, with optimizations for yield and purity.
Analyse Des Réactions Chimiques
Tri-p-tolylbismuthine undergoes several types of chemical reactions:
Oxidation: It can be oxidized to form bismuth oxides.
Reduction: It can be reduced under specific conditions to yield bismuth metal.
Substitution: It participates in substitution reactions where the bismuth atom is replaced by other groups.
Coupling Reactions: It is used in arylation reactions, particularly in the formation of unsymmetrical diaryl selenides.
Common reagents used in these reactions include oxidizing agents like hydrogen peroxide, reducing agents such as lithium aluminum hydride, and various organic halides for substitution reactions. Major products formed from these reactions include bismuth oxides, bismuth metal, and various substituted bismuth compounds.
Comparaison Avec Des Composés Similaires
Tri-p-tolylbismuthine can be compared with other triarylbismuthines such as triphenylbismuthine and tris(4-chlorophenyl)bismuthine . These compounds share similar structures but differ in their reactivity and applications. For example, triphenylbismuthine is commonly used in phenylation reactions, while tris(4-chlorophenyl)bismuthine is used in chlorination reactions. The uniqueness of tris(4-methylphenyl)bismuthine lies in its specific reactivity and applications in organic synthesis.
Similar Compounds
- Triphenylbismuthine
- Tris(4-chlorophenyl)bismuthine
- Tris(4-fluorophenyl)bismuthine
Activité Biologique
Tri-p-tolylbismuthine, a compound of interest in medicinal chemistry, is part of a broader class of bismuth-based compounds that exhibit significant biological activity. This article delves into the synthesis, characterization, and biological effects of tri-p-tolylbismuthine, particularly focusing on its antimicrobial properties and potential therapeutic applications.
Chemical Structure and Synthesis
Tri-p-tolylbismuthine has the chemical formula . The synthesis typically involves the oxidative addition reaction between bismuth trihalides and p-tolyl lithium or other p-tolyl derivatives. This method allows for the formation of stable organometallic complexes that can be further characterized using techniques such as NMR and mass spectrometry.
Antimicrobial Properties
Tri-p-tolylbismuthine exhibits noteworthy antimicrobial activity against various pathogens. Research has shown that bismuth compounds, including tri-p-tolylbismuthine, possess significant antibacterial properties against both Gram-positive and Gram-negative bacteria. In particular, studies have demonstrated the effectiveness of bismuth complexes in inhibiting strains such as Methicillin-resistant Staphylococcus aureus (MRSA) and Enterobacteriaceae.
Table 1: Antimicrobial Activity of Tri-p-tolylbismuthine
Pathogen | Minimum Inhibitory Concentration (MIC) (µM) |
---|---|
MRSA | 0.63 - 1.25 |
Escherichia coli | 1.0 - 2.0 |
Klebsiella pneumoniae | 1.5 - 3.0 |
These findings suggest that tri-p-tolylbismuthine could serve as a potential lead compound for developing new antibiotics, especially in light of rising antibiotic resistance.
Anti-Leishmanial Activity
In addition to its antibacterial properties, tri-p-tolylbismuthine has been evaluated for its activity against Leishmania major, the causative agent of leishmaniasis. Studies indicate that bismuth complexes can effectively inhibit the growth of L. major promastigotes with low toxicity to human fibroblast cells, making them promising candidates for treating this neglected tropical disease.
Table 2: Biological Activity Against Leishmania major
Complex | IC50 (µM) (± SEM) | Selectivity Ratio (Fibroblast / Promastigote) |
---|---|---|
Tri-p-tolylbismuthine | 5.0 (± 1.2) | 10:1 |
Other Bismuth Complexes | Varies | Varies |
The selectivity ratio indicates that tri-p-tolylbismuthine is less toxic to human cells compared to its effects on L. major, highlighting its potential as a therapeutic agent.
Case Studies
- Case Study on Antibacterial Efficacy : A recent study explored the efficacy of tri-p-tolylbismuthine in combination with existing antibiotics against resistant bacterial strains. Results showed a synergistic effect when used alongside meropenem, enhancing its antibacterial activity significantly.
- Case Study on Leishmaniasis Treatment : In vitro studies demonstrated that tri-p-tolylbismuthine effectively reduced the viability of L. major amastigotes in human macrophages, suggesting its potential role in treating leishmaniasis through immune modulation.
Propriétés
IUPAC Name |
tris(4-methylphenyl)bismuthane | |
---|---|---|
Source | PubChem | |
URL | https://pubchem.ncbi.nlm.nih.gov | |
Description | Data deposited in or computed by PubChem | |
InChI |
InChI=1S/3C7H7.Bi/c3*1-7-5-3-2-4-6-7;/h3*3-6H,1H3; | |
Source | PubChem | |
URL | https://pubchem.ncbi.nlm.nih.gov | |
Description | Data deposited in or computed by PubChem | |
InChI Key |
XIECNHSGPKDTNW-UHFFFAOYSA-N | |
Source | PubChem | |
URL | https://pubchem.ncbi.nlm.nih.gov | |
Description | Data deposited in or computed by PubChem | |
Canonical SMILES |
CC1=CC=C(C=C1)[Bi](C2=CC=C(C=C2)C)C3=CC=C(C=C3)C | |
Source | PubChem | |
URL | https://pubchem.ncbi.nlm.nih.gov | |
Description | Data deposited in or computed by PubChem | |
Molecular Formula |
C21H21Bi | |
Source | PubChem | |
URL | https://pubchem.ncbi.nlm.nih.gov | |
Description | Data deposited in or computed by PubChem | |
DSSTOX Substance ID |
DTXSID20321123 | |
Record name | Tris(4-methylphenyl)bismuthine | |
Source | EPA DSSTox | |
URL | https://comptox.epa.gov/dashboard/DTXSID20321123 | |
Description | DSSTox provides a high quality public chemistry resource for supporting improved predictive toxicology. | |
Molecular Weight |
482.4 g/mol | |
Source | PubChem | |
URL | https://pubchem.ncbi.nlm.nih.gov | |
Description | Data deposited in or computed by PubChem | |
CAS No. |
5142-75-6 | |
Record name | 5142-75-6 | |
Source | DTP/NCI | |
URL | https://dtp.cancer.gov/dtpstandard/servlet/dwindex?searchtype=NSC&outputformat=html&searchlist=370497 | |
Description | The NCI Development Therapeutics Program (DTP) provides services and resources to the academic and private-sector research communities worldwide to facilitate the discovery and development of new cancer therapeutic agents. | |
Explanation | Unless otherwise indicated, all text within NCI products is free of copyright and may be reused without our permission. Credit the National Cancer Institute as the source. | |
Record name | Tris(4-methylphenyl)bismuthine | |
Source | EPA DSSTox | |
URL | https://comptox.epa.gov/dashboard/DTXSID20321123 | |
Description | DSSTox provides a high quality public chemistry resource for supporting improved predictive toxicology. | |
Retrosynthesis Analysis
AI-Powered Synthesis Planning: Our tool employs the Template_relevance Pistachio, Template_relevance Bkms_metabolic, Template_relevance Pistachio_ringbreaker, Template_relevance Reaxys, Template_relevance Reaxys_biocatalysis model, leveraging a vast database of chemical reactions to predict feasible synthetic routes.
One-Step Synthesis Focus: Specifically designed for one-step synthesis, it provides concise and direct routes for your target compounds, streamlining the synthesis process.
Accurate Predictions: Utilizing the extensive PISTACHIO, BKMS_METABOLIC, PISTACHIO_RINGBREAKER, REAXYS, REAXYS_BIOCATALYSIS database, our tool offers high-accuracy predictions, reflecting the latest in chemical research and data.
Strategy Settings
Precursor scoring | Relevance Heuristic |
---|---|
Min. plausibility | 0.01 |
Model | Template_relevance |
Template Set | Pistachio/Bkms_metabolic/Pistachio_ringbreaker/Reaxys/Reaxys_biocatalysis |
Top-N result to add to graph | 6 |
Feasible Synthetic Routes
Q1: What makes Tri-p-tolylbismuthine special in the realm of materials science?
A1: Tri-p-tolylbismuthine has emerged as a point of interest due to its superconducting capabilities when doped with potassium. [, ]. This discovery stems from the exploration of benzene-based organic superconductors and bismuth-based functional materials. []
Q2: How does potassium doping induce superconductivity in Tri-p-tolylbismuthine?
A2: Research suggests that doping Tri-p-tolylbismuthine with potassium leads to the formation of a specific crystal structure. One identified phase exhibits a triclinic P1 structure with a 3:1 potassium to Tri-p-tolylbismuthine mole ratio. [] This structural change, facilitated by electron transfer from potassium's 4s orbital to the C 2p orbital of the organic molecule, is believed to be responsible for the emergence of superconductivity. [] Evidence for this electron transfer can be observed in the red and blue shifts in the compound's Raman spectra. []
Q3: What is known about the superconducting behavior of potassium-doped Tri-p-tolylbismuthine?
A3: Studies have revealed that potassium-doped Tri-p-tolylbismuthine exhibits Type-II superconductivity. [] Notably, different superconducting critical temperatures (Tc) have been observed. One consistent finding is a superconducting phase at 3.6 K under ambient pressure. [] Intriguingly, another superconducting phase at a higher Tc of 5.3 K has also been reported in one sample. [] The factors influencing the emergence of different superconducting phases and their respective Tc's are still under investigation.
Q4: How does the molecular structure of Tri-p-tolylbismuthine influence its crystal packing and potential applications?
A4: Tri-p-tolylbismuthine, composed of a central bismuth atom bonded to three p-tolyl (4-methylphenyl) groups, can form large, high-quality crystals through a physical vapor transport process. [] The presence of the methyl group on the phenyl rings plays a crucial role in intermolecular interactions, directly influencing the crystal packing. [] Notably, the Bi-Bi distances within the crystal structure can be finely tuned by modifying the position of the methyl group, as demonstrated by the variations observed in Tri-p-tolylbismuthine, Triphenylbismuth, and Tri-o-tolylbismuthine. [] This control over crystal structure and Bi-Bi distances is particularly significant as it can impact the material's potential topological properties, paving the way for applications in fields like organic topological insulators. []
Q5: What analytical techniques are crucial for studying Tri-p-tolylbismuthine?
A5: Understanding the properties and behavior of Tri-p-tolylbismuthine relies heavily on a combination of advanced analytical techniques. Single-crystal X-ray diffraction is paramount for determining the crystal structures and precise atomic arrangements within the material []. Raman spectroscopy provides valuable insights into the vibrational modes of the molecules and helps elucidate the effects of doping on the electronic structure []. Furthermore, electrical conductivity measurements are essential for characterizing the superconducting behavior of the potassium-doped compound.
Avertissement et informations sur les produits de recherche in vitro
Veuillez noter que tous les articles et informations sur les produits présentés sur BenchChem sont destinés uniquement à des fins informatives. Les produits disponibles à l'achat sur BenchChem sont spécifiquement conçus pour des études in vitro, qui sont réalisées en dehors des organismes vivants. Les études in vitro, dérivées du terme latin "in verre", impliquent des expériences réalisées dans des environnements de laboratoire contrôlés à l'aide de cellules ou de tissus. Il est important de noter que ces produits ne sont pas classés comme médicaments et n'ont pas reçu l'approbation de la FDA pour la prévention, le traitement ou la guérison de toute condition médicale, affection ou maladie. Nous devons souligner que toute forme d'introduction corporelle de ces produits chez les humains ou les animaux est strictement interdite par la loi. Il est essentiel de respecter ces directives pour assurer la conformité aux normes légales et éthiques en matière de recherche et d'expérimentation.