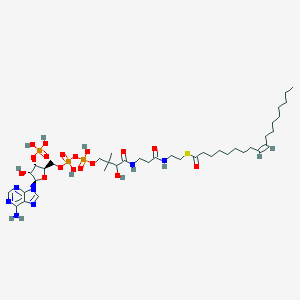
oleoyl-CoA
Vue d'ensemble
Description
Méthodes De Préparation
Voies de synthèse et conditions de réaction : L'oléoyl-CoA peut être synthétisé par estérification de l'acide oléique avec la coenzyme A. Cette réaction implique généralement l'activation de l'acide oléique en oléoyl-adénylate, suivie du transfert du groupe oléoyle à la coenzyme A. Les conditions de réaction comprennent souvent la présence d'ATP, d'ions magnésium et d'enzymes spécifiques telles que l'acyl-CoA synthétase .
Méthodes de production industrielle : Dans les milieux industriels, l'oléoyl-CoA est produit à l'aide de méthodes biotechnologiques impliquant des micro-organismes génétiquement modifiés. Ces micro-organismes sont conçus pour surexprimer les enzymes qui catalysent la formation d'oléoyl-CoA à partir de l'acide oléique et de la coenzyme A. Le processus de production est optimisé pour un rendement et une pureté élevés .
Types de réactions :
Réduction : Il peut être réduit pour former des dérivés d'acyl-CoA d'acides gras saturés.
Réactifs et conditions courantes :
Oxydation : Nécessite des enzymes telles que l'acyl-CoA déshydrogénase, l'énoyl-CoA hydratase et la β-hydroxyacyl-CoA déshydrogénase.
Réduction : Implique des réductases et du NADPH comme agent réducteur.
Substitution : Catalysé par les acyltransférases en présence de molécules acceptrices comme le glycérol ou le cholestérol.
Principaux produits :
Oxydation : Acétyl-CoA et dérivés d'acyl-CoA à chaîne plus courte.
Réduction : Acyl-CoA d'acides gras saturés.
Substitution : Monoacylglycérols, diacylglycérols, triacylglycérols et esters de cholestérol.
4. Applications de recherche scientifique
L'oléoyl-CoA a une large gamme d'applications dans la recherche scientifique :
Biologie : Joue un rôle dans l'étude des voies de signalisation lipidique et de la dynamique membranaire.
Médecine : La recherche sur l'oléoyl-CoA contribue à comprendre les troubles métaboliques tels que l'obésité, le diabète et les maladies cardiovasculaires.
Industrie : Utilisé dans la production de biocarburants et de plastiques biodégradables.
5. Mécanisme d'action
L'oléoyl-CoA exerce ses effets principalement par son rôle de donneur de groupe acyle dans diverses réactions biochimiques. Il est impliqué dans la synthèse de lipides complexes, y compris les phospholipides, les triglycérides et les esters de cholestérol. Les cibles moléculaires de l'oléoyl-CoA comprennent des enzymes telles que l'acyl-CoA : cholestérol acyltransférase, qui catalyse l'estérification du cholestérol, et la lysophosphatidylcholine acyltransférase, qui incorpore des groupes oléoyle dans les phospholipides .
Composés similaires :
Palmitoyl-CoA : Un dérivé d'acyl-CoA d'acide gras saturé impliqué dans des voies métaboliques similaires à celles de l'oléoyl-CoA.
Stéaroyl-CoA : Un autre acyl-CoA d'acide gras saturé qui sert de substrat à la désaturation pour former de l'oléoyl-CoA.
Linoléoyl-CoA : Un acyl-CoA d'acide gras insaturé impliqué dans la biosynthèse des acides gras polyinsaturés.
Unicité de l'oléoyl-CoA : L'oléoyl-CoA est unique en raison de son rôle de produit majeur de la stéaroyl-CoA désaturase 1, qui catalyse la conversion de la stéaroyl-CoA en oléoyl-CoA. Cette étape de désaturation est cruciale pour la biosynthèse des acides gras monoinsaturés, qui sont des composants essentiels des membranes cellulaires et des molécules de signalisation .
Applications De Recherche Scientifique
Role in Mycobacterial Survival
Oleoyl-CoA plays a significant role in the survival mechanisms of mycobacteria. Research indicates that it regulates the interaction of the transcriptional regulator RaaS with DNA, which is essential for mycobacterial persistence under stress conditions. In a study, this compound was shown to bind directly to RaaS, influencing gene expression related to mycobacterial efflux pumps and metabolic adaptation during growth phases. This binding was quantified with a dissociation constant () of approximately 3.65 μM, highlighting its potency as a regulatory molecule in bacterial metabolism .
Case Study: Mycobacterial Growth Regulation
- Objective : To understand how this compound affects mycobacterial gene expression.
- Method : Electrophoretic mobility shift assays (EMSAs) were used to assess the binding of this compound to RaaS.
- Findings : this compound completely inhibited RaaS-mediated band shifts at concentrations above 15 μM, indicating its strong regulatory role .
Modulation of K ATP Channels
This compound is also recognized for its ability to modulate ATP-sensitive potassium (K ATP) channels in cardiac and pancreatic tissues. Studies have demonstrated that this compound significantly reduces the ATP sensitivity of these channels, which is critical for maintaining cellular energy homeostasis. The modulation mechanism appears similar to that of phosphatidylinositol phosphates (PIPs), suggesting a shared pathway in lipid-mediated signaling .
Data Table: Effects of this compound on K ATP Channels
Channel Type | Initial (μM) | After this compound (μM) | Fold Change |
---|---|---|---|
Cardiac | 8 ± 0.4 | 1.4 ± 0.1 | 180 ± 12 |
Pancreatic | 18 ± 3 | 1.6 ± 0.3 | 99 ± 25 |
Industrial Applications
In industrial contexts, this compound derivatives are used in the synthesis of various bio-based lubricants and additives. For instance, estolides derived from oleic acid and other hydroxy fatty acids have been synthesized using immobilized lipases, showcasing this compound's utility in producing environmentally friendly lubricants with desirable properties like low viscosity and good thermal stability .
Case Study: Estolide Synthesis
- Objective : To synthesize estolides from oleic acid.
- Method : Utilization of immobilized lipases in solvent-free conditions.
- Results : The estolides exhibited excellent low-temperature properties and viscosity indices suitable for industrial applications .
Pharmacological Implications
This compound's influence extends into pharmacology, where it has been implicated in drug formulation strategies. For example, its role as a component in Pluronic lecithin organogels has been explored for enhancing the transdermal delivery of therapeutic agents like dexamethasone and ketoprofen. The presence of this compound improved the permeability profile of these drugs compared to traditional formulations .
Data Table: Drug Delivery Efficacy
Formulation Type | Drug Loaded | Permeability Improvement Factor |
---|---|---|
Pluronic Lecithin Organogel (Control) | Dexamethasone (0.5%) | Baseline |
Pluronic Lecithin Organogel (with this compound) | Ketoprofen (10%) | 3x |
Mécanisme D'action
Oleoyl-CoA exerts its effects primarily through its role as an acyl group donor in various biochemical reactions. It is involved in the synthesis of complex lipids, including phospholipids, triglycerides, and cholesterol esters. The molecular targets of this compound include enzymes such as acyl-CoA:cholesterol acyltransferase, which catalyzes the esterification of cholesterol, and lysophosphatidylcholine acyltransferase, which incorporates oleoyl groups into phospholipids .
Comparaison Avec Des Composés Similaires
Palmitoyl-CoA: A saturated fatty acyl-CoA derivative involved in similar metabolic pathways as oleoyl-CoA.
Stearoyl-CoA: Another saturated fatty acyl-CoA that serves as a substrate for desaturation to form this compound.
Linthis compound: An unsaturated fatty acyl-CoA involved in the biosynthesis of polyunsaturated fatty acids.
Uniqueness of this compound: this compound is unique due to its role as a major product of stearoyl-CoA desaturase 1, which catalyzes the conversion of stearoyl-CoA to this compound. This desaturation step is crucial for the biosynthesis of monounsaturated fatty acids, which are essential components of cell membranes and signaling molecules .
Propriétés
IUPAC Name |
S-[2-[3-[[4-[[[5-(6-aminopurin-9-yl)-4-hydroxy-3-phosphonooxyoxolan-2-yl]methoxy-hydroxyphosphoryl]oxy-hydroxyphosphoryl]oxy-2-hydroxy-3,3-dimethylbutanoyl]amino]propanoylamino]ethyl] octadec-9-enethioate | |
---|---|---|
Details | Computed by Lexichem TK 2.7.0 (PubChem release 2021.05.07) | |
Source | PubChem | |
URL | https://pubchem.ncbi.nlm.nih.gov | |
Description | Data deposited in or computed by PubChem | |
InChI |
InChI=1S/C39H68N7O17P3S/c1-4-5-6-7-8-9-10-11-12-13-14-15-16-17-18-19-30(48)67-23-22-41-29(47)20-21-42-37(51)34(50)39(2,3)25-60-66(57,58)63-65(55,56)59-24-28-33(62-64(52,53)54)32(49)38(61-28)46-27-45-31-35(40)43-26-44-36(31)46/h11-12,26-28,32-34,38,49-50H,4-10,13-25H2,1-3H3,(H,41,47)(H,42,51)(H,55,56)(H,57,58)(H2,40,43,44)(H2,52,53,54) | |
Details | Computed by InChI 1.0.6 (PubChem release 2021.05.07) | |
Source | PubChem | |
URL | https://pubchem.ncbi.nlm.nih.gov | |
Description | Data deposited in or computed by PubChem | |
InChI Key |
XDUHQPOXLUAVEE-UHFFFAOYSA-N | |
Details | Computed by InChI 1.0.6 (PubChem release 2021.05.07) | |
Source | PubChem | |
URL | https://pubchem.ncbi.nlm.nih.gov | |
Description | Data deposited in or computed by PubChem | |
Canonical SMILES |
CCCCCCCCC=CCCCCCCCC(=O)SCCNC(=O)CCNC(=O)C(C(C)(C)COP(=O)(O)OP(=O)(O)OCC1C(C(C(O1)N2C=NC3=C(N=CN=C32)N)O)OP(=O)(O)O)O | |
Details | Computed by OEChem 2.3.0 (PubChem release 2021.05.07) | |
Source | PubChem | |
URL | https://pubchem.ncbi.nlm.nih.gov | |
Description | Data deposited in or computed by PubChem | |
Molecular Formula |
C39H68N7O17P3S | |
Details | Computed by PubChem 2.1 (PubChem release 2021.05.07) | |
Source | PubChem | |
URL | https://pubchem.ncbi.nlm.nih.gov | |
Description | Data deposited in or computed by PubChem | |
Molecular Weight |
1032.0 g/mol | |
Details | Computed by PubChem 2.1 (PubChem release 2021.05.07) | |
Source | PubChem | |
URL | https://pubchem.ncbi.nlm.nih.gov | |
Description | Data deposited in or computed by PubChem | |
Retrosynthesis Analysis
AI-Powered Synthesis Planning: Our tool employs the Template_relevance Pistachio, Template_relevance Bkms_metabolic, Template_relevance Pistachio_ringbreaker, Template_relevance Reaxys, Template_relevance Reaxys_biocatalysis model, leveraging a vast database of chemical reactions to predict feasible synthetic routes.
One-Step Synthesis Focus: Specifically designed for one-step synthesis, it provides concise and direct routes for your target compounds, streamlining the synthesis process.
Accurate Predictions: Utilizing the extensive PISTACHIO, BKMS_METABOLIC, PISTACHIO_RINGBREAKER, REAXYS, REAXYS_BIOCATALYSIS database, our tool offers high-accuracy predictions, reflecting the latest in chemical research and data.
Strategy Settings
Precursor scoring | Relevance Heuristic |
---|---|
Min. plausibility | 0.01 |
Model | Template_relevance |
Template Set | Pistachio/Bkms_metabolic/Pistachio_ringbreaker/Reaxys/Reaxys_biocatalysis |
Top-N result to add to graph | 6 |
Feasible Synthetic Routes
A: Oleoyl-CoA, a long-chain acyl-coenzyme A ester, demonstrates diverse interactions within cellular systems. It activates ATP-sensitive potassium (KATP) channels in cardiac muscle cells [, ] by directly binding to the Kir6.2 subunit []. This interaction increases the open probability of these channels and impairs their inhibition by ATP []. Furthermore, this compound inhibits the glucose-6-phosphate transporter in oilseed rape plastids, indirectly reducing fatty acid synthesis due to ATP deprivation []. It also plays a crucial role in lipid metabolism, serving as a substrate for enzymes involved in triacylglycerol synthesis [, , , ] and phosphatidic acid formation [, , ].
ANone: The provided research focuses primarily on the biological activity and metabolic roles of this compound, with limited information on its material compatibility and stability in non-biological contexts.
A: this compound is not a catalyst itself, but it plays a crucial role as a substrate for various enzymes. For instance, it is a substrate for this compound:1-acylglycerophosphocholine O-acyltransferase in mouse brain microsomes, contributing to phospholipid synthesis []. This compound is also a substrate for desaturases, specifically the Δ12- and Δ6-desaturases involved in the biosynthesis of γ-linolenic acid in borage seeds [].
ANone: The provided research papers do not delve into the computational chemistry or modeling of this compound.
A: Comparing this compound with an analogue, oleoyl-(1, N6-etheno)-CoA, revealed the importance of the adenine moiety for citrate synthase inhibition []. Oleoyl-(1, N6-etheno)-CoA, despite being a better detergent, exhibited weaker inhibition and binding, suggesting a specific interaction between the adenine of this compound and the enzyme []. This highlights the significance of specific structural elements for this compound's biological activity. Additionally, research on the impact of different fatty acyl-CoA chain lengths on enzyme activity reveals that this compound (C18:1) shows higher activity with certain enzymes compared to shorter chain acyl-CoAs [, , , ].
ANone: The available research does not cover the pharmacokinetics or pharmacodynamics of this compound. Studies primarily focus on its biochemical roles and interactions within cellular systems.
A: While the provided papers do not discuss therapeutic applications or efficacy of this compound, they do demonstrate its biological activity in various in vitro and ex vivo experimental settings. For instance, this compound inhibits citrate synthase activity in vitro [], modulates KATP channel activity in excised membrane patches [, ], and affects fatty acid synthesis in isolated plastid preparations [, ].
Avertissement et informations sur les produits de recherche in vitro
Veuillez noter que tous les articles et informations sur les produits présentés sur BenchChem sont destinés uniquement à des fins informatives. Les produits disponibles à l'achat sur BenchChem sont spécifiquement conçus pour des études in vitro, qui sont réalisées en dehors des organismes vivants. Les études in vitro, dérivées du terme latin "in verre", impliquent des expériences réalisées dans des environnements de laboratoire contrôlés à l'aide de cellules ou de tissus. Il est important de noter que ces produits ne sont pas classés comme médicaments et n'ont pas reçu l'approbation de la FDA pour la prévention, le traitement ou la guérison de toute condition médicale, affection ou maladie. Nous devons souligner que toute forme d'introduction corporelle de ces produits chez les humains ou les animaux est strictement interdite par la loi. Il est essentiel de respecter ces directives pour assurer la conformité aux normes légales et éthiques en matière de recherche et d'expérimentation.