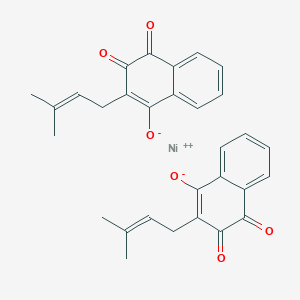
Nickel lapachol
Vue d'ensemble
Description
Nickel lapachol is a coordination complex formed between nickel and lapachol, a naturally occurring 1,4-naphthoquinone. Lapachol is derived from the bark of the Tabebuia tree and has been studied for its various pharmacological properties, including anticancer, antimicrobial, and anti-inflammatory activities . The complexation with nickel enhances the biological activities of lapachol, making this compound a compound of significant interest in medicinal chemistry and pharmacology .
Applications De Recherche Scientifique
Nickel lapachol has a wide range of scientific research applications:
Chemistry: It is used as a catalyst in various organic reactions, including cross-coupling and radical reactions.
Biology: The compound has shown potential as an antimicrobial and antifungal agent.
Medicine: this compound exhibits significant anticancer activity, particularly against glioma and breast cancer cells
Mécanisme D'action
The mechanisms of action underlying the observed effects of naphthoquinone derivatives like lapachol are mainly due to their capacity to interact with topoisomerases and to generate semiquinone radicals and reactive oxygen species (ROS) inside the cell . Hypoxic mammalian cells become more sensitive to the toxic action of cisplatin when also treated with nickel lapachol .
Safety and Hazards
Nickel and its compounds have hazard classifications, and it is important that they are handled and used correctly in the workplace to ensure that health risks to workers are eliminated . Adequate understanding of risks and hazards from exposures to nickel during production and use, coupled with various jurisdictional and international regulations on nickel exposure, means there is increased protection of workers and consumers from the toxicological effects associated with nickel exposure .
Orientations Futures
Méthodes De Préparation
Synthetic Routes and Reaction Conditions: Nickel lapachol can be synthesized through the coordination of lapachol with nickel salts. A common method involves dissolving lapachol in an appropriate solvent, such as ethanol, and then adding a nickel salt, such as nickel chloride or nickel acetate. The reaction mixture is stirred at room temperature or slightly elevated temperatures to facilitate the formation of the complex .
Industrial Production Methods: While specific industrial production methods for this compound are not well-documented, the general approach would involve scaling up the laboratory synthesis. This would include optimizing reaction conditions, such as temperature, solvent, and concentration, to maximize yield and purity. The use of continuous flow reactors and automated systems could further enhance the efficiency of industrial production.
Analyse Des Réactions Chimiques
Types of Reactions: Nickel lapachol undergoes various chemical reactions, including:
Oxidation: The complex can participate in oxidation reactions, where the naphthoquinone moiety is oxidized to form more reactive species.
Reduction: this compound can be reduced under specific conditions, leading to the formation of reduced naphthoquinone derivatives.
Substitution: The complex can undergo substitution reactions where ligands in the coordination sphere of nickel are replaced by other ligands.
Common Reagents and Conditions:
Oxidation: Common oxidizing agents include hydrogen peroxide and potassium permanganate.
Reduction: Reducing agents such as sodium borohydride or lithium aluminum hydride are used.
Substitution: Ligands like phosphines or amines can be used to replace existing ligands in the complex.
Major Products Formed: The major products formed from these reactions depend on the specific conditions and reagents used. For example, oxidation can lead to the formation of quinone derivatives, while reduction can yield hydroquinone derivatives .
Comparaison Avec Des Composés Similaires
- Iron lapachol
- Copper lapachol
- Zinc lapachol
- Indium lapachol
Propriétés
IUPAC Name |
2-(3-methylbut-2-enyl)-3,4-dioxonaphthalen-1-olate;nickel(2+) | |
---|---|---|
Source | PubChem | |
URL | https://pubchem.ncbi.nlm.nih.gov | |
Description | Data deposited in or computed by PubChem | |
InChI |
InChI=1S/2C15H14O3.Ni/c2*1-9(2)7-8-12-13(16)10-5-3-4-6-11(10)14(17)15(12)18;/h2*3-7,16H,8H2,1-2H3;/q;;+2/p-2 | |
Source | PubChem | |
URL | https://pubchem.ncbi.nlm.nih.gov | |
Description | Data deposited in or computed by PubChem | |
InChI Key |
KYSJTXUSOJUYHX-UHFFFAOYSA-L | |
Source | PubChem | |
URL | https://pubchem.ncbi.nlm.nih.gov | |
Description | Data deposited in or computed by PubChem | |
Canonical SMILES |
CC(=CCC1=C(C2=CC=CC=C2C(=O)C1=O)[O-])C.CC(=CCC1=C(C2=CC=CC=C2C(=O)C1=O)[O-])C.[Ni+2] | |
Source | PubChem | |
URL | https://pubchem.ncbi.nlm.nih.gov | |
Description | Data deposited in or computed by PubChem | |
Molecular Formula |
C30H26NiO6 | |
Source | PubChem | |
URL | https://pubchem.ncbi.nlm.nih.gov | |
Description | Data deposited in or computed by PubChem | |
DSSTOX Substance ID |
DTXSID40925985 | |
Record name | Nickel(2+) bis[2-(3-methylbut-2-en-1-yl)-3,4-dioxo-3,4-dihydronaphthalen-1-olate] | |
Source | EPA DSSTox | |
URL | https://comptox.epa.gov/dashboard/DTXSID40925985 | |
Description | DSSTox provides a high quality public chemistry resource for supporting improved predictive toxicology. | |
Molecular Weight |
541.2 g/mol | |
Source | PubChem | |
URL | https://pubchem.ncbi.nlm.nih.gov | |
Description | Data deposited in or computed by PubChem | |
CAS No. |
127796-52-5 | |
Record name | Nickel lapachol | |
Source | ChemIDplus | |
URL | https://pubchem.ncbi.nlm.nih.gov/substance/?source=chemidplus&sourceid=0127796525 | |
Description | ChemIDplus is a free, web search system that provides access to the structure and nomenclature authority files used for the identification of chemical substances cited in National Library of Medicine (NLM) databases, including the TOXNET system. | |
Record name | Nickel(2+) bis[2-(3-methylbut-2-en-1-yl)-3,4-dioxo-3,4-dihydronaphthalen-1-olate] | |
Source | EPA DSSTox | |
URL | https://comptox.epa.gov/dashboard/DTXSID40925985 | |
Description | DSSTox provides a high quality public chemistry resource for supporting improved predictive toxicology. | |
Retrosynthesis Analysis
AI-Powered Synthesis Planning: Our tool employs the Template_relevance Pistachio, Template_relevance Bkms_metabolic, Template_relevance Pistachio_ringbreaker, Template_relevance Reaxys, Template_relevance Reaxys_biocatalysis model, leveraging a vast database of chemical reactions to predict feasible synthetic routes.
One-Step Synthesis Focus: Specifically designed for one-step synthesis, it provides concise and direct routes for your target compounds, streamlining the synthesis process.
Accurate Predictions: Utilizing the extensive PISTACHIO, BKMS_METABOLIC, PISTACHIO_RINGBREAKER, REAXYS, REAXYS_BIOCATALYSIS database, our tool offers high-accuracy predictions, reflecting the latest in chemical research and data.
Strategy Settings
Precursor scoring | Relevance Heuristic |
---|---|
Min. plausibility | 0.01 |
Model | Template_relevance |
Template Set | Pistachio/Bkms_metabolic/Pistachio_ringbreaker/Reaxys/Reaxys_biocatalysis |
Top-N result to add to graph | 6 |
Feasible Synthetic Routes
Avertissement et informations sur les produits de recherche in vitro
Veuillez noter que tous les articles et informations sur les produits présentés sur BenchChem sont destinés uniquement à des fins informatives. Les produits disponibles à l'achat sur BenchChem sont spécifiquement conçus pour des études in vitro, qui sont réalisées en dehors des organismes vivants. Les études in vitro, dérivées du terme latin "in verre", impliquent des expériences réalisées dans des environnements de laboratoire contrôlés à l'aide de cellules ou de tissus. Il est important de noter que ces produits ne sont pas classés comme médicaments et n'ont pas reçu l'approbation de la FDA pour la prévention, le traitement ou la guérison de toute condition médicale, affection ou maladie. Nous devons souligner que toute forme d'introduction corporelle de ces produits chez les humains ou les animaux est strictement interdite par la loi. Il est essentiel de respecter ces directives pour assurer la conformité aux normes légales et éthiques en matière de recherche et d'expérimentation.