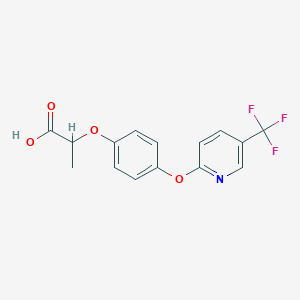
Fluazifop
Vue d'ensemble
Description
Molecular Structure Analysis
Fluazifop-inducible glutathione transferases from Phaseolus vulgaris (PvGSTs) catalyze a broad range of reactions and exhibit quite varied substrate specificity . Molecular modeling and structural analysis was used to identify key structural characteristics and to provide insights into the substrate specificity and the catalytic mechanism of these enzymes .Chemical Reactions Analysis
Fluazifop-P-butyl is rapidly hydrolyzed by esterases and the main metabolite is its acid derivative fluazifop . The residues are extracted with acidified organic solvent, the esters and conjugates are hydrolyzed with 6 M hydrochloric acid, then the extracts are cleaned-up by solid phase extraction .Physical And Chemical Properties Analysis
Fluazifop-P-butyl is a systemic, post-emergence herbicide that selectively controls both annual and perennial grass weeds in non-graminaceous crops . It is rapidly hydrolyzed by esterases and the main metabolite is its acid derivative fluazifop . The half-life of fluazifop-P-butyl in soils is one to two weeks .Applications De Recherche Scientifique
Agricultural Herbicide
Fluazifop is widely used as a post-emergence herbicide for the control of grasses in broad-leaved crops. It’s particularly effective against annual and perennial grasses in crops like cotton, soybeans, tree nuts, asparagus, and coffee. The herbicide is often used in combination with crop oil concentrate or nonionic surfactants to enhance its efficacy .
Enhancing Plant Growth
Studies have shown that the application of Fluazifop can positively influence plant growth by controlling weeds such as Johnsongrass, which competes with crops for nutrients and sunlight .
Irrigation Application
Fluazifop can be applied through sprinkler irrigation systems, which has been found to control large crabgrass as effectively as conventional spray applications .
Selective Weed Control
The herbicide is selective and has systemic activity in grass weeds while being safe for use around non-target plants like P. vulgaris (common bean). This selectivity is due to its inhibition of the enzyme acetyl-coenzyme A carboxylase (ACCase), which is essential for lipid synthesis in grasses .
Pest Management in Fruit Crops
Fluazifop-P is used as a herbicide in pome fruit orchards, providing effective weed control without harming the fruit trees .
Invasive Species Control
Applications of Fluazifop have been successful in controlling invasive grass species such as quackgrass and jubatagrass, which can outcompete native plants and disrupt ecosystems .
Propriétés
IUPAC Name |
2-[4-[5-(trifluoromethyl)pyridin-2-yl]oxyphenoxy]propanoic acid | |
---|---|---|
Source | PubChem | |
URL | https://pubchem.ncbi.nlm.nih.gov | |
Description | Data deposited in or computed by PubChem | |
InChI |
InChI=1S/C15H12F3NO4/c1-9(14(20)21)22-11-3-5-12(6-4-11)23-13-7-2-10(8-19-13)15(16,17)18/h2-9H,1H3,(H,20,21) | |
Source | PubChem | |
URL | https://pubchem.ncbi.nlm.nih.gov | |
Description | Data deposited in or computed by PubChem | |
InChI Key |
YUVKUEAFAVKILW-UHFFFAOYSA-N | |
Source | PubChem | |
URL | https://pubchem.ncbi.nlm.nih.gov | |
Description | Data deposited in or computed by PubChem | |
Canonical SMILES |
CC(C(=O)O)OC1=CC=C(C=C1)OC2=NC=C(C=C2)C(F)(F)F | |
Source | PubChem | |
URL | https://pubchem.ncbi.nlm.nih.gov | |
Description | Data deposited in or computed by PubChem | |
Molecular Formula |
C15H12F3NO4 | |
Source | PubChem | |
URL | https://pubchem.ncbi.nlm.nih.gov | |
Description | Data deposited in or computed by PubChem | |
DSSTOX Substance ID |
DTXSID3058163 | |
Record name | Fluazifop | |
Source | EPA DSSTox | |
URL | https://comptox.epa.gov/dashboard/DTXSID3058163 | |
Description | DSSTox provides a high quality public chemistry resource for supporting improved predictive toxicology. | |
Molecular Weight |
327.25 g/mol | |
Source | PubChem | |
URL | https://pubchem.ncbi.nlm.nih.gov | |
Description | Data deposited in or computed by PubChem | |
Product Name |
Fluazifop | |
CAS RN |
69335-91-7 | |
Record name | Fluazifop | |
Source | CAS Common Chemistry | |
URL | https://commonchemistry.cas.org/detail?cas_rn=69335-91-7 | |
Description | CAS Common Chemistry is an open community resource for accessing chemical information. Nearly 500,000 chemical substances from CAS REGISTRY cover areas of community interest, including common and frequently regulated chemicals, and those relevant to high school and undergraduate chemistry classes. This chemical information, curated by our expert scientists, is provided in alignment with our mission as a division of the American Chemical Society. | |
Explanation | The data from CAS Common Chemistry is provided under a CC-BY-NC 4.0 license, unless otherwise stated. | |
Record name | Fluazifop [ANSI:BSI:ISO] | |
Source | ChemIDplus | |
URL | https://pubchem.ncbi.nlm.nih.gov/substance/?source=chemidplus&sourceid=0069335917 | |
Description | ChemIDplus is a free, web search system that provides access to the structure and nomenclature authority files used for the identification of chemical substances cited in National Library of Medicine (NLM) databases, including the TOXNET system. | |
Record name | Fluazifop | |
Source | EPA DSSTox | |
URL | https://comptox.epa.gov/dashboard/DTXSID3058163 | |
Description | DSSTox provides a high quality public chemistry resource for supporting improved predictive toxicology. | |
Record name | FLUAZIFOP | |
Source | FDA Global Substance Registration System (GSRS) | |
URL | https://gsrs.ncats.nih.gov/ginas/app/beta/substances/8JD49YW45K | |
Description | The FDA Global Substance Registration System (GSRS) enables the efficient and accurate exchange of information on what substances are in regulated products. Instead of relying on names, which vary across regulatory domains, countries, and regions, the GSRS knowledge base makes it possible for substances to be defined by standardized, scientific descriptions. | |
Explanation | Unless otherwise noted, the contents of the FDA website (www.fda.gov), both text and graphics, are not copyrighted. They are in the public domain and may be republished, reprinted and otherwise used freely by anyone without the need to obtain permission from FDA. Credit to the U.S. Food and Drug Administration as the source is appreciated but not required. | |
Synthesis routes and methods I
Procedure details
Synthesis routes and methods II
Procedure details
Retrosynthesis Analysis
AI-Powered Synthesis Planning: Our tool employs the Template_relevance Pistachio, Template_relevance Bkms_metabolic, Template_relevance Pistachio_ringbreaker, Template_relevance Reaxys, Template_relevance Reaxys_biocatalysis model, leveraging a vast database of chemical reactions to predict feasible synthetic routes.
One-Step Synthesis Focus: Specifically designed for one-step synthesis, it provides concise and direct routes for your target compounds, streamlining the synthesis process.
Accurate Predictions: Utilizing the extensive PISTACHIO, BKMS_METABOLIC, PISTACHIO_RINGBREAKER, REAXYS, REAXYS_BIOCATALYSIS database, our tool offers high-accuracy predictions, reflecting the latest in chemical research and data.
Strategy Settings
Precursor scoring | Relevance Heuristic |
---|---|
Min. plausibility | 0.01 |
Model | Template_relevance |
Template Set | Pistachio/Bkms_metabolic/Pistachio_ringbreaker/Reaxys/Reaxys_biocatalysis |
Top-N result to add to graph | 6 |
Feasible Synthetic Routes
Q & A
A: Fluazifop-butyl is a pro-herbicide that is rapidly hydrolyzed to fluazifop acid, the active form, within plants. Fluazifop acid inhibits acetyl-CoA carboxylase (ACCase), a key enzyme in fatty acid biosynthesis. [, , , , ] This inhibition disrupts lipid synthesis, particularly in actively growing regions like meristems, rhizomes, and stolons of grasses. [, , ] The disruption of lipid synthesis ultimately leads to plant death. [, , ]
A: * Molecular formula: C19H17F3N2O4* Molecular weight: 394.35 g/mol* Spectroscopic data: Spectroscopic data for fluazifop-butyl and its metabolite, fluazifop acid, are detailed in several papers using techniques like High-Performance Liquid Chromatography (HPLC) and liquid scintillation spectrometry. [, , , , ]
A: Fluazifop-butyl activity is influenced by various environmental factors. Low temperatures, low humidity, and water stress have been shown to reduce its efficacy. [] Conversely, high post-treatment temperature and humidity enhance its activity. [] Rain shortly after application can reduce its performance due to wash-off. [] Studies have also investigated the effect of environmental conditions on epicuticular wax deposition in giant foxtail, which can influence fluazifop-P absorption. [] Drought stress and low temperatures increased leaf wax content, while low light intensity decreased it. [] These changes in wax composition can alter leaf wettability and herbicide uptake. []
A: Fluazifop-butyl degrades primarily through microbial metabolism and hydrolysis in the environment. [] It has a soil half-life of one to two weeks and is not readily degraded by sunlight. [] While selective for grasses, potential impacts on non-target organisms and its persistence in the environment require careful consideration. Research on strategies to mitigate negative impacts is crucial. [, ]
A: Fluazifop-butyl is typically formulated as an emulsifiable concentrate. [] Its formulation and stability can be influenced by factors such as adjuvant type, temperature, and storage conditions. [, , , ] Studies have explored different formulations, including seed treatments and combinations with other herbicides, to improve efficacy and target specific weed species. [, ]
A: Analytical methods employed for the determination of fluazifop-butyl and its metabolite, fluazifop acid, include:* High-Performance Liquid Chromatography (HPLC): This technique separates and quantifies the herbicide and its metabolite in plant tissues and other matrices. [, , , , ] * Liquid scintillation spectrometry: This method utilizes radiolabeled fluazifop-butyl to track its uptake, translocation, and metabolism in plants. [, , , ]* Liquid chromatography/tandem mass spectrometry (LC/MS/MS): This powerful technique allows for identification and quantification of fluazifop-P-butyl metabolites in resistant weed biotypes. []
Avertissement et informations sur les produits de recherche in vitro
Veuillez noter que tous les articles et informations sur les produits présentés sur BenchChem sont destinés uniquement à des fins informatives. Les produits disponibles à l'achat sur BenchChem sont spécifiquement conçus pour des études in vitro, qui sont réalisées en dehors des organismes vivants. Les études in vitro, dérivées du terme latin "in verre", impliquent des expériences réalisées dans des environnements de laboratoire contrôlés à l'aide de cellules ou de tissus. Il est important de noter que ces produits ne sont pas classés comme médicaments et n'ont pas reçu l'approbation de la FDA pour la prévention, le traitement ou la guérison de toute condition médicale, affection ou maladie. Nous devons souligner que toute forme d'introduction corporelle de ces produits chez les humains ou les animaux est strictement interdite par la loi. Il est essentiel de respecter ces directives pour assurer la conformité aux normes légales et éthiques en matière de recherche et d'expérimentation.