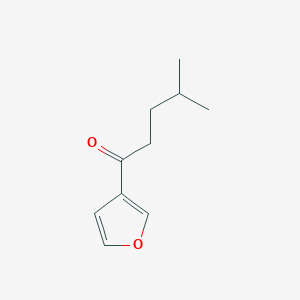
Perilla cétone
Vue d'ensemble
Description
La perillacétone est un composé terpénoïde naturel que l'on trouve dans l'huile essentielle de Perilla frutescens, communément appelée menthe pourpre. Elle se compose d'un cycle furanique avec une chaîne latérale à six carbones contenant un groupe fonctionnel cétone. Cette huile incolore est sensible à l'oxygène et se colore en vieillissant . La perillacétone a été identifiée pour la première fois en 1943 par Sebe comme le principal composant de l'huile essentielle de Perilla frutescens .
Applications De Recherche Scientifique
Perillaketone has a wide range of scientific research applications:
Chemistry: Used as a starting material for the synthesis of various organic compounds.
Biology: Studied for its effects on cellular processes and enzyme activities.
Industry: Utilized in the production of fragrances and flavoring agents.
Mécanisme D'action
Target of Action
Perilla ketone, a natural terpenoid found in Perilla frutescens, has been shown to interact with several targets. One of the primary targets is the CYP4B1 enzyme . This enzyme plays a crucial role in the metabolism of xenobiotics and endogenous compounds . Another key target is the AKT1 gene, which plays a critical role in multiple cellular processes such as glucose metabolism, apoptosis, cell proliferation, transcription, and cell migration .
Mode of Action
Perilla ketone’s interaction with its targets leads to various physiological changes. For instance, the difference in the mechanism of CYP4B1 enzyme action on perilla ketone and on 4-ipomeanol may explain why perilla ketone has a greater ability to induce apoptosis . Furthermore, perilla ketone may increase pulmonary microvascular permeability, thus inducing toxicity .
Biochemical Pathways
Perilla ketone affects several biochemical pathways. For instance, it has been found to regulate apoptosis, T cell receptor, and PI3K-AKT signaling pathways . These pathways play a crucial role in cell survival, growth, and proliferation.
Pharmacokinetics
It’s known that perilla ketone is a component of the essential oil of perilla frutescens, suggesting that it may be absorbed and distributed throughout the body via the circulatory system .
Result of Action
The molecular and cellular effects of perilla ketone’s action are diverse. It has been shown to have promising antitumor, antifungal, anti-inflammatory, and other activities . Specifically, perilla ketone induces interstitial pneumonia, severe respiratory distress, and death in animals, such as cattle and sheep .
Action Environment
Environmental factors can influence the action, efficacy, and stability of perilla ketone. For instance, the morphological and physiological response of Perilla frutescens, the plant from which perilla ketone is derived, changes with climatic and edaphic factors with altitude . This suggests that the production and efficacy of perilla ketone may also be influenced by these environmental conditions.
Analyse Biochimique
Biochemical Properties
Perilla ketone interacts with various enzymes and proteins. For instance, it has been found to interact with a novel double bond reductase (PfDBRs), which are members of the NADPH-dependent, medium-chain dehydrogenase/reductase (MDR) superfamily . PfDBRs catalyze the conversion of isoegoma ketone and egoma ketone into Perilla ketone in in vitro enzymatic assays .
Cellular Effects
Perilla ketone has been shown to have promising antitumor, antifungal, antirheumatoid arthritis, antiobesity, anti-inflammatory, healing-promoting, and other activities . It can be used to combat toxicity from immunotherapy . When cattle and horses consume purple mint when grazing in fields in which it grows, the Perilla ketone causes pulmonary edema leading to a condition sometimes called perilla mint toxicosis .
Molecular Mechanism
The molecular mechanism of Perilla ketone involves its interaction with the CYP4B1 enzyme. The difference in the mechanism of CYP4B1 enzyme action on Perilla ketone and on 4-ipomeanol may explain why Perilla ketone has a greater ability to induce apoptosis than the positive control drug, 4-ipomeanol .
Temporal Effects in Laboratory Settings
Early studies on Perilla ketone focused on the toxicity of Perilla ketone in animals; specifically, Perilla ketone induces interstitial pneumonia, severe respiratory distress, and death in animals, such as cattle and sheep .
Dosage Effects in Animal Models
Perilla ketone, from the essential oil of Perilla frutescens, is a pulmonary edemagenic agent for laboratory animals and livestock, when they take a high amount of P. frutescens .
Metabolic Pathways
Perilla ketone is involved in several metabolic pathways. Based on transcriptome data, several genes and transcription factors were predicted to be associated with monoterpenoid biosynthesis, and their expression profiles were positively correlated with variations in Perilla ketone abundance, suggesting their potential functions in Perilla ketone biosynthesis .
Méthodes De Préparation
Voies synthétiques et conditions de réaction
La perillacétone a été synthétisée pour la première fois en 1957 par Matsuura à partir du chlorure de 3-furoyle et d'un composé organocadmium similaire au réactif de Gilman obtenu à partir d'un réactif de Grignard d'isoamyle et de chlorure de cadmium . Une autre méthode implique la réaction de Stille, où un composé 3-furyl-organoétain réagit avec le chlorure d'isocaproyle dans un solvant de tétrahydrofurane, ce qui donne de la perillacétone avec un rendement de 74 % .
Méthodes de production industrielle
Bien que les méthodes de production industrielle spécifiques de la perillacétone ne soient pas bien documentées, les voies synthétiques mentionnées ci-dessus peuvent être mises à l'échelle pour les applications industrielles. La réaction de Stille, en particulier, est une méthode polyvalente qui peut être adaptée à la production à grande échelle.
Analyse Des Réactions Chimiques
Types de réactions
La perillacétone subit plusieurs types de réactions chimiques, notamment :
Oxydation : Le cycle furanique peut être époxydé.
Réduction : Le groupe fonctionnel cétone peut être réduit en alcool.
Substitution : Le cycle furanique peut subir des réactions de substitution.
Réactifs et conditions courants
Oxydation : L'époxydation du cycle furanique peut être réalisée en utilisant des peracides ou du peroxyde d'hydrogène.
Réduction : La réduction du groupe cétone peut être réalisée en utilisant des agents réducteurs comme le borohydrure de sodium ou l'hydrure de lithium et d'aluminium.
Substitution : Les réactions de substitution sur le cycle furanique peuvent être effectuées en utilisant des électrophiles dans des conditions acides ou basiques.
Principaux produits formés
Époxydation : Dérivés époxy de la perillacétone.
Réduction : Dérivés alcooliques de la perillacétone.
Substitution : Divers dérivés furaniques substitués.
Applications de la recherche scientifique
La perillacétone a une large gamme d'applications de recherche scientifique :
Chimie : Utilisée comme matière première pour la synthèse de divers composés organiques.
Biologie : Étudiée pour ses effets sur les processus cellulaires et les activités enzymatiques.
Industrie : Utilisée dans la production de parfums et d'arômes.
Mécanisme d'action
La perillacétone exerce ses effets par le biais de plusieurs cibles moléculaires et voies. Elle peut subir une époxydation ou une hydroxylation sous l'action de l'enzyme CYP4B1, conduisant à la formation de métabolites réactifs . Ces métabolites peuvent interagir avec les composants cellulaires, augmentant la perméabilité microvasculaire pulmonaire et induisant une toxicité . Le mécanisme d'action exact peut varier en fonction du contexte biologique spécifique.
Comparaison Avec Des Composés Similaires
Composés similaires
Isoegomaketone : Un autre composé trouvé dans Perilla frutescens avec une structure chimique et des propriétés similaires.
Cétone d'egoma : Un composé apparenté avec des voies biosynthétiques similaires.
Pulégone : Une cétone monoterpénique avec des groupes fonctionnels similaires.
Unicité
La perillacétone est unique en raison de sa combinaison spécifique d'un cycle furanique et d'une chaîne latérale à six carbones avec un groupe fonctionnel cétone. Cette structure confère une réactivité chimique et une activité biologique distinctes, ce qui en fait un composé précieux pour diverses applications.
Activité Biologique
Perilla ketone, a bioactive compound derived from the essential oil of Perilla frutescens, has garnered attention for its diverse biological activities and potential therapeutic applications. This article explores the pharmacological properties, mechanisms of action, and relevant case studies associated with perilla ketone.
Overview of Perilla Ketone
Perilla ketone is primarily found in Perilla frutescens, a plant widely used in traditional medicine and culinary practices, especially in East Asia. It is recognized for its potent biological activities, including anti-inflammatory, antitumor, and antimicrobial effects.
Pharmacological Activities
Recent studies have highlighted a range of biological activities attributed to perilla ketone:
- Antitumor Activity : Perilla ketone exhibits significant cytotoxic effects against various cancer cell lines. Research indicates that it can induce apoptosis in cancer cells and inhibit tumor growth by modulating signaling pathways involved in cell proliferation and survival .
- Anti-inflammatory Effects : The compound has been shown to reduce inflammation by inhibiting pro-inflammatory cytokines such as TNF-α and IL-6. This is particularly relevant in conditions like rheumatoid arthritis and other inflammatory disorders .
- Antimicrobial Properties : Perilla ketone demonstrates antibacterial and antifungal activities, making it a candidate for developing natural preservatives and therapeutic agents against infections .
- Neuroprotective Effects : Studies suggest that perilla ketone may protect neurons from oxidative stress and neuroinflammation, potentially offering benefits in neurodegenerative diseases .
The biological activities of perilla ketone can be attributed to several mechanisms:
- Modulation of Signaling Pathways : Perilla ketone influences various signaling pathways, including the NF-kB pathway, which is crucial for regulating inflammation and immune responses. By inhibiting this pathway, perilla ketone can reduce the expression of inflammatory mediators .
- Oxidative Stress Reduction : The compound enhances antioxidant defenses by increasing the expression of antioxidant enzymes, thereby mitigating oxidative damage in cells .
- Apoptosis Induction : In cancer cells, perilla ketone triggers apoptosis through intrinsic pathways involving mitochondrial dysfunction and caspase activation .
Case Studies
Several studies have documented the effects of perilla ketone in both animal models and clinical settings:
- Animal Model of IgA Nephropathy :
- Cancer Cell Lines :
- Inflammatory Disorders :
Data Summary
The following table summarizes key findings related to the biological activity of perilla ketone:
Biological Activity | Mechanism of Action | Model/Study Type | Outcome |
---|---|---|---|
Antitumor | Apoptosis induction | Cancer cell lines | Significant growth inhibition |
Anti-inflammatory | NF-kB pathway inhibition | Animal models | Reduced cytokine levels |
Antimicrobial | Direct bacterial/fungal inhibition | In vitro studies | Effective against pathogens |
Neuroprotective | Oxidative stress mitigation | Neurodegenerative models | Improved neuronal survival |
Renal protective | Reduction of proteinuria | Animal model (IgA nephropathy) | Statistically significant improvement |
Propriétés
IUPAC Name |
1-(furan-3-yl)-4-methylpentan-1-one | |
---|---|---|
Source | PubChem | |
URL | https://pubchem.ncbi.nlm.nih.gov | |
Description | Data deposited in or computed by PubChem | |
InChI |
InChI=1S/C10H14O2/c1-8(2)3-4-10(11)9-5-6-12-7-9/h5-8H,3-4H2,1-2H3 | |
Source | PubChem | |
URL | https://pubchem.ncbi.nlm.nih.gov | |
Description | Data deposited in or computed by PubChem | |
InChI Key |
LVHLZMUFIYAEQB-UHFFFAOYSA-N | |
Source | PubChem | |
URL | https://pubchem.ncbi.nlm.nih.gov | |
Description | Data deposited in or computed by PubChem | |
Canonical SMILES |
CC(C)CCC(=O)C1=COC=C1 | |
Source | PubChem | |
URL | https://pubchem.ncbi.nlm.nih.gov | |
Description | Data deposited in or computed by PubChem | |
Molecular Formula |
C10H14O2 | |
Source | PubChem | |
URL | https://pubchem.ncbi.nlm.nih.gov | |
Description | Data deposited in or computed by PubChem | |
DSSTOX Substance ID |
DTXSID10203828 | |
Record name | Perilla ketone | |
Source | EPA DSSTox | |
URL | https://comptox.epa.gov/dashboard/DTXSID10203828 | |
Description | DSSTox provides a high quality public chemistry resource for supporting improved predictive toxicology. | |
Molecular Weight |
166.22 g/mol | |
Source | PubChem | |
URL | https://pubchem.ncbi.nlm.nih.gov | |
Description | Data deposited in or computed by PubChem | |
Boiling Point |
223.00 to 225.00 °C. @ 760.00 mm Hg | |
Record name | Perilla ketone | |
Source | Human Metabolome Database (HMDB) | |
URL | http://www.hmdb.ca/metabolites/HMDB0035328 | |
Description | The Human Metabolome Database (HMDB) is a freely available electronic database containing detailed information about small molecule metabolites found in the human body. | |
Explanation | HMDB is offered to the public as a freely available resource. Use and re-distribution of the data, in whole or in part, for commercial purposes requires explicit permission of the authors and explicit acknowledgment of the source material (HMDB) and the original publication (see the HMDB citing page). We ask that users who download significant portions of the database cite the HMDB paper in any resulting publications. | |
CAS No. |
553-84-4 | |
Record name | 1-(3-Furyl)-4-methyl-1-pentanone | |
Source | CAS Common Chemistry | |
URL | https://commonchemistry.cas.org/detail?cas_rn=553-84-4 | |
Description | CAS Common Chemistry is an open community resource for accessing chemical information. Nearly 500,000 chemical substances from CAS REGISTRY cover areas of community interest, including common and frequently regulated chemicals, and those relevant to high school and undergraduate chemistry classes. This chemical information, curated by our expert scientists, is provided in alignment with our mission as a division of the American Chemical Society. | |
Explanation | The data from CAS Common Chemistry is provided under a CC-BY-NC 4.0 license, unless otherwise stated. | |
Record name | Perilla ketone | |
Source | ChemIDplus | |
URL | https://pubchem.ncbi.nlm.nih.gov/substance/?source=chemidplus&sourceid=0000553844 | |
Description | ChemIDplus is a free, web search system that provides access to the structure and nomenclature authority files used for the identification of chemical substances cited in National Library of Medicine (NLM) databases, including the TOXNET system. | |
Record name | Perilla ketone | |
Source | DTP/NCI | |
URL | https://dtp.cancer.gov/dtpstandard/servlet/dwindex?searchtype=NSC&outputformat=html&searchlist=348407 | |
Description | The NCI Development Therapeutics Program (DTP) provides services and resources to the academic and private-sector research communities worldwide to facilitate the discovery and development of new cancer therapeutic agents. | |
Explanation | Unless otherwise indicated, all text within NCI products is free of copyright and may be reused without our permission. Credit the National Cancer Institute as the source. | |
Record name | Perilla ketone | |
Source | EPA DSSTox | |
URL | https://comptox.epa.gov/dashboard/DTXSID10203828 | |
Description | DSSTox provides a high quality public chemistry resource for supporting improved predictive toxicology. | |
Record name | PERILLA KETONE | |
Source | FDA Global Substance Registration System (GSRS) | |
URL | https://gsrs.ncats.nih.gov/ginas/app/beta/substances/CV69S6Y94V | |
Description | The FDA Global Substance Registration System (GSRS) enables the efficient and accurate exchange of information on what substances are in regulated products. Instead of relying on names, which vary across regulatory domains, countries, and regions, the GSRS knowledge base makes it possible for substances to be defined by standardized, scientific descriptions. | |
Explanation | Unless otherwise noted, the contents of the FDA website (www.fda.gov), both text and graphics, are not copyrighted. They are in the public domain and may be republished, reprinted and otherwise used freely by anyone without the need to obtain permission from FDA. Credit to the U.S. Food and Drug Administration as the source is appreciated but not required. | |
Record name | Perilla ketone | |
Source | Human Metabolome Database (HMDB) | |
URL | http://www.hmdb.ca/metabolites/HMDB0035328 | |
Description | The Human Metabolome Database (HMDB) is a freely available electronic database containing detailed information about small molecule metabolites found in the human body. | |
Explanation | HMDB is offered to the public as a freely available resource. Use and re-distribution of the data, in whole or in part, for commercial purposes requires explicit permission of the authors and explicit acknowledgment of the source material (HMDB) and the original publication (see the HMDB citing page). We ask that users who download significant portions of the database cite the HMDB paper in any resulting publications. | |
Synthesis routes and methods
Procedure details
Retrosynthesis Analysis
AI-Powered Synthesis Planning: Our tool employs the Template_relevance Pistachio, Template_relevance Bkms_metabolic, Template_relevance Pistachio_ringbreaker, Template_relevance Reaxys, Template_relevance Reaxys_biocatalysis model, leveraging a vast database of chemical reactions to predict feasible synthetic routes.
One-Step Synthesis Focus: Specifically designed for one-step synthesis, it provides concise and direct routes for your target compounds, streamlining the synthesis process.
Accurate Predictions: Utilizing the extensive PISTACHIO, BKMS_METABOLIC, PISTACHIO_RINGBREAKER, REAXYS, REAXYS_BIOCATALYSIS database, our tool offers high-accuracy predictions, reflecting the latest in chemical research and data.
Strategy Settings
Precursor scoring | Relevance Heuristic |
---|---|
Min. plausibility | 0.01 |
Model | Template_relevance |
Template Set | Pistachio/Bkms_metabolic/Pistachio_ringbreaker/Reaxys/Reaxys_biocatalysis |
Top-N result to add to graph | 6 |
Feasible Synthetic Routes
A: Perilla ketone increases pulmonary microvascular permeability, leading to protein-rich fluid leakage into lung tissues. [, , ] This results in pulmonary edema, compromised gas exchange, and respiratory distress. [, , , ] The exact mechanism of action at the cellular level remains unclear, but evidence suggests direct damage to microvascular endothelial cells and Type I pneumocytes. [, ]
A: Perilla ketone exposure leads to an influx of neutrophils into the lungs and increased levels of proinflammatory cytokines. [, ] Histological examinations reveal a marked proliferation of Type II pneumocytes in the lungs of affected animals. []
ANone: Perilla ketone (1-(3-furyl)-4-methylpentan-1-one) is a 3-acylfuran. It consists of a furan ring substituted at the 3-position with an isoamyl ketone group.
ANone: The molecular formula of perilla ketone is C10H14O2, and its molecular weight is 166.22 g/mol.
ANone: This specific aspect is not addressed in the provided research papers.
ANone: This aspect is not covered in the provided research papers.
ANone: No computational studies or QSAR models related to perilla ketone are discussed in the provided research papers.
A: Research suggests that bulky substituents on the furan ring can decrease the toxicity of 3-furyl ketones. For example, compounds like 3-furylphenyl ketone, 3-furylphenethyl ketone, and 1-3-furyl-4,4-dimethylpentan-1-one exhibited reduced toxicity compared to perilla ketone in mice. [] This suggests steric hindrance of bioactivation or alternative metabolic pathways might play a role. []
ANone: Information regarding specific formulation strategies or stability studies is not available in the provided research papers.
ANone: The provided research articles do not specifically discuss SHE regulations for perilla ketone.
A: Various animal models, including sheep, mice, hamsters, rabbits, dogs, and pigs, have been utilized to investigate perilla ketone toxicity. [, , , , , ] Sheep models have been particularly valuable in studying perilla ketone-induced pulmonary edema, mimicking aspects of Acute Respiratory Distress Syndrome (ARDS) in humans. [, , ]
A: Yes, in vitro studies using bovine aortic endothelial cells demonstrated that perilla ketone directly increases endothelial cell monolayer permeability, suggesting a direct effect on endothelial cells. []
ANone: Information on resistance or cross-resistance mechanisms related to perilla ketone is not found in the provided research papers.
A: Perilla ketone is a potent lung toxin in several animal species, causing acute pulmonary edema, emphysema, and respiratory distress. [, , , , , , ]
ANone: The provided research articles do not discuss drug delivery or targeting strategies for perilla ketone.
ANone: Researchers have employed various techniques for perilla ketone analysis, including:
- High-speed countercurrent chromatography (HSCCC): For preparative separation and purification of perilla ketone from plant material. []
- Gas chromatography-mass spectrometry (GC-MS): Widely used for identification and quantification of perilla ketone in plant extracts and essential oils. [, , , , , , ]
- High-performance liquid chromatography (HPLC): Used to determine perilla ketone concentrations in plant material, particularly to study the variation in content across different parts and growth stages. []
ANone: The provided research papers primarily focus on perilla ketone toxicity in animals and do not offer information regarding its environmental impact or degradation pathways.
ANone: This aspect is not explored in detail in the provided research papers.
ANone: Specific details regarding analytical method validation for perilla ketone are not provided in the research papers.
ANone: The provided research papers do not focus on quality control and assurance measures for perilla ketone.
ANone: The potential immunogenicity of perilla ketone is not discussed in the provided research papers.
ANone: Information on perilla ketone's interactions with drug transporters is not available in the provided research.
A: While some research suggests a potential role for cytochrome P450 enzymes in perilla ketone bioactivation, findings are not conclusive. [, ] For example, in vitro studies with bovine aortic endothelial cells showed that the cytochrome P450 inhibitor ketoconazole did not prevent the permeability-increasing effects of perilla ketone, suggesting alternative mechanisms might be involved. []
ANone: The provided research primarily focuses on perilla ketone toxicity and does not provide specific information regarding its biocompatibility or biodegradability.
ANone: The research papers do not directly compare perilla ketone to alternative compounds or discuss substitutes.
ANone: This aspect is not addressed in the provided research papers.
ANone: The provided research papers do not specifically discuss research infrastructure or resources dedicated to perilla ketone.
A: Perilla ketone was identified as a potent lung toxin in the 1970s. Early research linked perilla ketone to atypical interstitial pneumonia in livestock consuming Perilla frutescens. [, ]
ANone: Understanding perilla ketone toxicity contributes to:
- Veterinary medicine: Identifying and managing perilla ketone poisoning in livestock. [, , , ]
- Human health: Assessing potential risks associated with perilla ketone exposure through dietary sources and traditional medicine. []
- Pulmonary research: Perilla ketone serves as a valuable tool in animal models for investigating the mechanisms of acute lung injury and pulmonary edema. [, , ]
Avertissement et informations sur les produits de recherche in vitro
Veuillez noter que tous les articles et informations sur les produits présentés sur BenchChem sont destinés uniquement à des fins informatives. Les produits disponibles à l'achat sur BenchChem sont spécifiquement conçus pour des études in vitro, qui sont réalisées en dehors des organismes vivants. Les études in vitro, dérivées du terme latin "in verre", impliquent des expériences réalisées dans des environnements de laboratoire contrôlés à l'aide de cellules ou de tissus. Il est important de noter que ces produits ne sont pas classés comme médicaments et n'ont pas reçu l'approbation de la FDA pour la prévention, le traitement ou la guérison de toute condition médicale, affection ou maladie. Nous devons souligner que toute forme d'introduction corporelle de ces produits chez les humains ou les animaux est strictement interdite par la loi. Il est essentiel de respecter ces directives pour assurer la conformité aux normes légales et éthiques en matière de recherche et d'expérimentation.