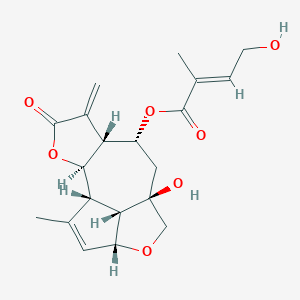
Eupalinilide C
Vue d'ensemble
Description
Eupalinilide C is a naturally occurring sesquiterpene lactone isolated from the plant Eupatorium lindleyanum. This compound has garnered significant interest due to its potential therapeutic applications, particularly in the fields of medicine and pharmacy. This compound is known for its structural stability and ability to interact with various biological targets, making it a promising candidate for drug development .
Applications De Recherche Scientifique
Eupalinilide C has been widely studied for its potential therapeutic applications due to its ability to interact with various biological targets, such as G-protein coupled receptors, ion channels, and enzymes . Its applications include:
Chemistry: this compound serves as a valuable compound for studying sesquiterpene lactone chemistry and developing new synthetic methodologies.
Biology: The compound’s ability to modulate biological pathways makes it a useful tool for studying cellular processes and signaling pathways.
Industry: The compound’s structural stability and bioactivity make it a candidate for developing new pharmaceuticals and therapeutic agents.
Mécanisme D'action
Target of Action
Eupalinilide C is a naturally occurring cyclic peptide isolated from the marine sponge Eupatorium lindleyanum . It has been widely studied for its potential therapeutic applications, due to its ability to interact with various biological targets, such as G-protein coupled receptors, ion channels, and enzymes .
Mode of Action
This compound, like other sesquiterpene lactones, is a Michael addition acceptor . Its electrophilic moiety can react with the nucleophilic residues of the relevant active site, leading to a variety of bioactivities . This interaction with its targets results in changes at the molecular level, which can lead to various downstream effects.
Biochemical Pathways
It is known that sesquiterpene lactones, like this compound, can effectively inhibit tnbc cells in vitro . This suggests that this compound may affect pathways related to cell growth and proliferation, particularly in the context of cancer cells.
Pharmacokinetics
It is known that this compound is soluble in chloroform, ethyl acetate, dimethylsulfoxide and other solvents, but insoluble in water . This could potentially impact its absorption, distribution, metabolism, and excretion (ADME) properties, and thus its bioavailability.
Result of Action
The result of this compound’s action is largely dependent on the specific biological target it interacts with. For example, when interacting with cancer cells, it has been shown to have cytotoxic effects . More research is needed to fully understand the molecular and cellular effects of this compound’s action.
Action Environment
The action of this compound can be influenced by various environmental factors. could affect its efficacy and stability in different environments. Furthermore, its structural stability makes it a promising candidate for drug development .
Analyse Biochimique
Biochemical Properties
Eupalinilide C has been studied for its potential therapeutic applications due to its ability to interact with various biological targets, such as G-protein coupled receptors, ion channels, and enzymes . Its structural stability makes it a promising candidate for drug development .
Cellular Effects
For instance, Eupalinilide E was assessed for ex-vivo expansion activity on hematopoietic stem cells (HSCs) from human cord blood (CB) CD34+ cells in serum-free, SCF, TPO and FL stimulated 7-day cultures .
Molecular Mechanism
It is known that this compound and other similar sesquiterpene lactones are Michael addition acceptors, whose electrophilic moiety could react with the nucleophilic residues of the relevant active site and produce a variety of bioactivities .
Temporal Effects in Laboratory Settings
It is known that Eupalinilide E ex-vivo enhanced phenotyped (p) HSCs and glycolysis of CD34+ cells isolated 7 days after culture as measured by extracellular acidification rate .
Méthodes De Préparation
Synthetic Routes and Reaction Conditions: The synthesis of Eupalinilide C involves multiple steps, including late-stage allylic oxidations and chemoselective reduction and oxidation reactions. One successful synthetic strategy relied on late-stage allylic oxidations at two separate positions of the molecule, significantly increasing the breadth of reactions that could be used . Adaptations of existing chemical transformations, such as a modified Luche reduction and a selective homoallylic alcohol epoxidation, were required to achieve the desired product .
Industrial Production Methods: While specific industrial production methods for this compound are not well-documented, the scalable synthesis of similar compounds suggests that industrial production would likely involve optimizing the laboratory synthesis routes for larger-scale operations. This would include ensuring the availability of starting materials, optimizing reaction conditions, and implementing efficient purification processes.
Analyse Des Réactions Chimiques
Types of Reactions: Eupalinilide C undergoes various chemical reactions, including:
Oxidation: Late-stage allylic oxidations are crucial in the synthesis of this compound.
Reduction: Chemoselective reduction reactions are employed to achieve the desired stereochemistry.
Substitution: The compound can undergo nucleophilic substitution reactions due to its electrophilic moieties.
Common Reagents and Conditions:
Oxidation: Reagents such as oxidizing agents (e.g., m-chloroperbenzoic acid) are used for allylic oxidations.
Reduction: Modified Luche reduction conditions are employed to achieve selective reduction.
Substitution: Nucleophilic reagents can react with the electrophilic centers of this compound.
Major Products: The major products formed from these reactions include various oxidized and reduced derivatives of this compound, which can be further utilized in medicinal chemistry research.
Comparaison Avec Des Composés Similaires
Eupalinilide C is part of a class of sesquiterpene lactones that includes similar compounds such as Eupalinilide E, Eupachinilide B, and Eupachiilide A . These compounds share structural similarities but differ in their specific biological activities and therapeutic potentials. For example:
Eupalinilide E: Known for its selective cytotoxicity against certain cancer cell lines.
Eupachinilide B: Demonstrates potent cytotoxicity and has been studied for its anti-cancer properties.
Eupachiilide A: Exhibits significant bioactivity and has been investigated for its potential in treating triple-negative breast cancer.
This compound stands out due to its unique structural features and broad range of biological targets, making it a versatile compound for scientific research and drug development.
Propriétés
IUPAC Name |
[(1R,2R,6R,7R,9R,12R,15S)-9-hydroxy-14-methyl-5-methylidene-4-oxo-3,11-dioxatetracyclo[7.5.1.02,6.012,15]pentadec-13-en-7-yl] (E)-4-hydroxy-2-methylbut-2-enoate | |
---|---|---|
Source | PubChem | |
URL | https://pubchem.ncbi.nlm.nih.gov | |
Description | Data deposited in or computed by PubChem | |
InChI |
InChI=1S/C20H24O7/c1-9(4-5-21)18(22)26-13-7-20(24)8-25-12-6-10(2)14(16(12)20)17-15(13)11(3)19(23)27-17/h4,6,12-17,21,24H,3,5,7-8H2,1-2H3/b9-4+/t12-,13-,14+,15-,16-,17-,20+/m1/s1 | |
Source | PubChem | |
URL | https://pubchem.ncbi.nlm.nih.gov | |
Description | Data deposited in or computed by PubChem | |
InChI Key |
QWNQZWRFKIHTPL-NPUYJLKLSA-N | |
Source | PubChem | |
URL | https://pubchem.ncbi.nlm.nih.gov | |
Description | Data deposited in or computed by PubChem | |
Canonical SMILES |
CC1=CC2C3C1C4C(C(CC3(CO2)O)OC(=O)C(=CCO)C)C(=C)C(=O)O4 | |
Source | PubChem | |
URL | https://pubchem.ncbi.nlm.nih.gov | |
Description | Data deposited in or computed by PubChem | |
Isomeric SMILES |
CC1=C[C@@H]2[C@@H]3[C@H]1[C@@H]4[C@@H]([C@@H](C[C@@]3(CO2)O)OC(=O)/C(=C/CO)/C)C(=C)C(=O)O4 | |
Source | PubChem | |
URL | https://pubchem.ncbi.nlm.nih.gov | |
Description | Data deposited in or computed by PubChem | |
Molecular Formula |
C20H24O7 | |
Source | PubChem | |
URL | https://pubchem.ncbi.nlm.nih.gov | |
Description | Data deposited in or computed by PubChem | |
Molecular Weight |
376.4 g/mol | |
Source | PubChem | |
URL | https://pubchem.ncbi.nlm.nih.gov | |
Description | Data deposited in or computed by PubChem | |
Retrosynthesis Analysis
AI-Powered Synthesis Planning: Our tool employs the Template_relevance Pistachio, Template_relevance Bkms_metabolic, Template_relevance Pistachio_ringbreaker, Template_relevance Reaxys, Template_relevance Reaxys_biocatalysis model, leveraging a vast database of chemical reactions to predict feasible synthetic routes.
One-Step Synthesis Focus: Specifically designed for one-step synthesis, it provides concise and direct routes for your target compounds, streamlining the synthesis process.
Accurate Predictions: Utilizing the extensive PISTACHIO, BKMS_METABOLIC, PISTACHIO_RINGBREAKER, REAXYS, REAXYS_BIOCATALYSIS database, our tool offers high-accuracy predictions, reflecting the latest in chemical research and data.
Strategy Settings
Precursor scoring | Relevance Heuristic |
---|---|
Min. plausibility | 0.01 |
Model | Template_relevance |
Template Set | Pistachio/Bkms_metabolic/Pistachio_ringbreaker/Reaxys/Reaxys_biocatalysis |
Top-N result to add to graph | 6 |
Feasible Synthetic Routes
Avertissement et informations sur les produits de recherche in vitro
Veuillez noter que tous les articles et informations sur les produits présentés sur BenchChem sont destinés uniquement à des fins informatives. Les produits disponibles à l'achat sur BenchChem sont spécifiquement conçus pour des études in vitro, qui sont réalisées en dehors des organismes vivants. Les études in vitro, dérivées du terme latin "in verre", impliquent des expériences réalisées dans des environnements de laboratoire contrôlés à l'aide de cellules ou de tissus. Il est important de noter que ces produits ne sont pas classés comme médicaments et n'ont pas reçu l'approbation de la FDA pour la prévention, le traitement ou la guérison de toute condition médicale, affection ou maladie. Nous devons souligner que toute forme d'introduction corporelle de ces produits chez les humains ou les animaux est strictement interdite par la loi. Il est essentiel de respecter ces directives pour assurer la conformité aux normes légales et éthiques en matière de recherche et d'expérimentation.