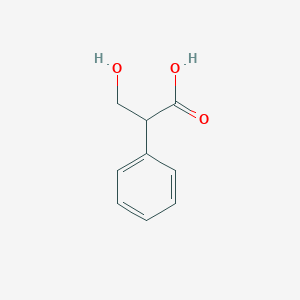
Tropic acid
Vue d'ensemble
Description
Tropic acid, also known as 2-Phenylhydracrylic acid, Tropate, or alpha-(Hydroxymethyl)phenylacetic acid, is a laboratory reagent used in the chemical synthesis of atropine and hyoscyamine . It is a chiral substance, existing as either a racemic mixture or as a single enantiomer .
Synthesis Analysis
Tropic acid can be synthesized from hydratropic acid and propionic acid . The process for preparing tropic acid comprises reacting sodium phenylacetate with magnesium and isopropyl chloride, reacting the resulting sodium salt of alpha-carboxybenzylmagnesium chloride with formaldehyde, and hydrolyzing the resulting complex .Molecular Structure Analysis
The molecular formula of Tropic Acid is C9H10O3 . It has a molecular weight of 166.17 . The structure of Tropic Acid is also available as a 2D Mol file .Chemical Reactions Analysis
Tropic acid is used in the synthesis of (−)-anisodine, atropine, and hyoscyamine . It undergoes a three-step decarboxylation and hydrolysis reactions to form Putrescine which gets methylated and undergo oxidative deamination to form N-methyl-Δ1-pyrrolinium .Physical And Chemical Properties Analysis
Tropic acid is a white to off-white crystalline powder . It has a melting point of 116-118 °C and a density of 1.1097 . It is soluble in water (20 g/L at 20 ºC), alcohol, ether, and slightly in benzene .Applications De Recherche Scientifique
Synthesis of Atropine and Hyoscyamine
Tropic acid is a laboratory reagent used in the chemical synthesis of atropine and hyoscyamine . These are two important alkaloids found in the Solanaceae family of plants, which have been used for centuries in medicine for their anticholinergic properties.
Esterification of Carboxylic Acids and Alcohols
Tropic acid can be used as a substrate in the esterification of carboxylic acids and alcohols in the presence of dried Dowex H+/NaI catalyst system . This process is crucial in the production of esters, which are widely used in a variety of industries, including the food, fragrance, and pharmaceutical industries.
Multistep Synthesis of Cyclic Pentadepsipeptides
Tropic acid can also be used in the multistep synthesis of cyclic pentadepsipeptides . These compounds have been found to have a variety of biological activities, including antimicrobial, antiviral, and anticancer properties.
Synthesis of Isocoumarin Derivatives
Tropic acid is used in the synthesis of an isocoumarin derivative by reacting with tetracyanobenzene (TCNB) via multicomponent photo reaction . Isocoumarins are a class of organic compounds that have been found to have a variety of biological activities, including antibacterial, antifungal, and anticancer properties.
Synthesis of (−)-Anisodine
Tropic acid is used in the synthesis of (−)-anisodine , a drug that has been used in China for the treatment of acute circulatory disorders.
Unique Synthesis and Isomerization
Tropic acid has a branching carbon skeleton and must originate either from the isomerization of a phenylpropionoid moiety or through a unique synthesis . This unique property of tropic acid makes it a valuable reagent in the study of organic chemistry and the synthesis of complex organic molecules.
Safety and Hazards
Tropic acid can cause skin irritation, serious eye irritation, and may cause respiratory irritation . It is recommended to avoid dust formation, avoid breathing mist, gas or vapors, avoid contacting with skin and eye, use personal protective equipment, wear chemical impermeable gloves, ensure adequate ventilation, and remove all sources of ignition .
Mécanisme D'action
Target of Action
Tropic acid is a key component in the synthesis of certain anticholinergic drugs, such as atropine and scopolamine . These drugs primarily target muscarinic acetylcholine receptors (mAChR) . These receptors play a crucial role in various functions of the nervous system, including smooth muscle contraction, heart rate regulation, and glandular secretion .
Mode of Action
When it is used to synthesize drugs like atropine, the resulting compound acts as anon-selective antagonist of muscarinic acetylcholine receptors . This means that these drugs bind to mAChR and block their activation by acetylcholine, a neurotransmitter. This blockade inhibits the parasympathetic nerve impulses, leading to effects such as pupil dilation (mydriasis) and paralysis of the eye’s ciliary muscle (cycloplegia) .
Biochemical Pathways
The biochemical pathways involving tropic acid primarily concern its role in the synthesis of anticholinergic drugs. For instance, tropic acid reacts with tropine to produce atropine . Similarly, it reacts with scopine in a Fischer esterification process to produce scopolamine . These reactions are part of larger biochemical pathways involving the metabolism and action of these drugs.
Pharmacokinetics
For example, atropine, a drug synthesized from tropic acid, is well absorbed orally and reaches significant levels in the central nervous system within 30-60 minutes . It has a two-phase elimination process with a rapid half-life of 2 hours and a slower half-life of 13 hours . It undergoes hepatic metabolism and subsequent renal excretion .
Result of Action
The primary result of the action of drugs synthesized from tropic acid, such as atropine, is the blockade of muscarinic acetylcholine receptors. This leads to various physiological effects, including dilation of the pupil and paralysis of the ciliary muscle in the eye . These effects are useful in diagnostic procedures involving the eye .
Action Environment
The action of drugs synthesized from tropic acid can be influenced by various environmental factors. For instance, the absorption, distribution, metabolism, and excretion of these drugs can be affected by factors such as the individual’s age, liver function, and the presence of other drugs . .
Propriétés
IUPAC Name |
3-hydroxy-2-phenylpropanoic acid | |
---|---|---|
Source | PubChem | |
URL | https://pubchem.ncbi.nlm.nih.gov | |
Description | Data deposited in or computed by PubChem | |
InChI |
InChI=1S/C9H10O3/c10-6-8(9(11)12)7-4-2-1-3-5-7/h1-5,8,10H,6H2,(H,11,12) | |
Source | PubChem | |
URL | https://pubchem.ncbi.nlm.nih.gov | |
Description | Data deposited in or computed by PubChem | |
InChI Key |
JACRWUWPXAESPB-UHFFFAOYSA-N | |
Source | PubChem | |
URL | https://pubchem.ncbi.nlm.nih.gov | |
Description | Data deposited in or computed by PubChem | |
Canonical SMILES |
C1=CC=C(C=C1)C(CO)C(=O)O | |
Source | PubChem | |
URL | https://pubchem.ncbi.nlm.nih.gov | |
Description | Data deposited in or computed by PubChem | |
Molecular Formula |
C9H10O3 | |
Source | PubChem | |
URL | https://pubchem.ncbi.nlm.nih.gov | |
Description | Data deposited in or computed by PubChem | |
DSSTOX Substance ID |
DTXSID90862179 | |
Record name | (+/-)-2-Phenyl-3-hydroxypropionic acid | |
Source | EPA DSSTox | |
URL | https://comptox.epa.gov/dashboard/DTXSID90862179 | |
Description | DSSTox provides a high quality public chemistry resource for supporting improved predictive toxicology. | |
Molecular Weight |
166.17 g/mol | |
Source | PubChem | |
URL | https://pubchem.ncbi.nlm.nih.gov | |
Description | Data deposited in or computed by PubChem | |
Product Name |
Tropic acid | |
CAS RN |
552-63-6, 529-64-6 | |
Record name | (±)-Tropic acid | |
Source | CAS Common Chemistry | |
URL | https://commonchemistry.cas.org/detail?cas_rn=552-63-6 | |
Description | CAS Common Chemistry is an open community resource for accessing chemical information. Nearly 500,000 chemical substances from CAS REGISTRY cover areas of community interest, including common and frequently regulated chemicals, and those relevant to high school and undergraduate chemistry classes. This chemical information, curated by our expert scientists, is provided in alignment with our mission as a division of the American Chemical Society. | |
Explanation | The data from CAS Common Chemistry is provided under a CC-BY-NC 4.0 license, unless otherwise stated. | |
Record name | Tropic acid | |
Source | ChemIDplus | |
URL | https://pubchem.ncbi.nlm.nih.gov/substance/?source=chemidplus&sourceid=0000552636 | |
Description | ChemIDplus is a free, web search system that provides access to the structure and nomenclature authority files used for the identification of chemical substances cited in National Library of Medicine (NLM) databases, including the TOXNET system. | |
Record name | TROPIC ACID | |
Source | DTP/NCI | |
URL | https://dtp.cancer.gov/dtpstandard/servlet/dwindex?searchtype=NSC&outputformat=html&searchlist=20990 | |
Description | The NCI Development Therapeutics Program (DTP) provides services and resources to the academic and private-sector research communities worldwide to facilitate the discovery and development of new cancer therapeutic agents. | |
Explanation | Unless otherwise indicated, all text within NCI products is free of copyright and may be reused without our permission. Credit the National Cancer Institute as the source. | |
Record name | Benzeneacetic acid, .alpha.-(hydroxymethyl)- | |
Source | EPA Chemicals under the TSCA | |
URL | https://www.epa.gov/chemicals-under-tsca | |
Description | EPA Chemicals under the Toxic Substances Control Act (TSCA) collection contains information on chemicals and their regulations under TSCA, including non-confidential content from the TSCA Chemical Substance Inventory and Chemical Data Reporting. | |
Record name | (+/-)-2-Phenyl-3-hydroxypropionic acid | |
Source | EPA DSSTox | |
URL | https://comptox.epa.gov/dashboard/DTXSID90862179 | |
Description | DSSTox provides a high quality public chemistry resource for supporting improved predictive toxicology. | |
Record name | (±)-(hydroxymethyl)phenylacetic acid | |
Source | European Chemicals Agency (ECHA) | |
URL | https://echa.europa.eu/substance-information/-/substanceinfo/100.008.201 | |
Description | The European Chemicals Agency (ECHA) is an agency of the European Union which is the driving force among regulatory authorities in implementing the EU's groundbreaking chemicals legislation for the benefit of human health and the environment as well as for innovation and competitiveness. | |
Explanation | Use of the information, documents and data from the ECHA website is subject to the terms and conditions of this Legal Notice, and subject to other binding limitations provided for under applicable law, the information, documents and data made available on the ECHA website may be reproduced, distributed and/or used, totally or in part, for non-commercial purposes provided that ECHA is acknowledged as the source: "Source: European Chemicals Agency, http://echa.europa.eu/". Such acknowledgement must be included in each copy of the material. ECHA permits and encourages organisations and individuals to create links to the ECHA website under the following cumulative conditions: Links can only be made to webpages that provide a link to the Legal Notice page. | |
Record name | Tropic acid | |
Source | European Chemicals Agency (ECHA) | |
URL | https://echa.europa.eu/substance-information/-/substanceinfo/100.007.697 | |
Description | The European Chemicals Agency (ECHA) is an agency of the European Union which is the driving force among regulatory authorities in implementing the EU's groundbreaking chemicals legislation for the benefit of human health and the environment as well as for innovation and competitiveness. | |
Explanation | Use of the information, documents and data from the ECHA website is subject to the terms and conditions of this Legal Notice, and subject to other binding limitations provided for under applicable law, the information, documents and data made available on the ECHA website may be reproduced, distributed and/or used, totally or in part, for non-commercial purposes provided that ECHA is acknowledged as the source: "Source: European Chemicals Agency, http://echa.europa.eu/". Such acknowledgement must be included in each copy of the material. ECHA permits and encourages organisations and individuals to create links to the ECHA website under the following cumulative conditions: Links can only be made to webpages that provide a link to the Legal Notice page. | |
Record name | TROPIC ACID | |
Source | FDA Global Substance Registration System (GSRS) | |
URL | https://gsrs.ncats.nih.gov/ginas/app/beta/substances/9RM4U80765 | |
Description | The FDA Global Substance Registration System (GSRS) enables the efficient and accurate exchange of information on what substances are in regulated products. Instead of relying on names, which vary across regulatory domains, countries, and regions, the GSRS knowledge base makes it possible for substances to be defined by standardized, scientific descriptions. | |
Explanation | Unless otherwise noted, the contents of the FDA website (www.fda.gov), both text and graphics, are not copyrighted. They are in the public domain and may be republished, reprinted and otherwise used freely by anyone without the need to obtain permission from FDA. Credit to the U.S. Food and Drug Administration as the source is appreciated but not required. | |
Record name | Tropate | |
Source | Human Metabolome Database (HMDB) | |
URL | http://www.hmdb.ca/metabolites/HMDB0062590 | |
Description | The Human Metabolome Database (HMDB) is a freely available electronic database containing detailed information about small molecule metabolites found in the human body. | |
Explanation | HMDB is offered to the public as a freely available resource. Use and re-distribution of the data, in whole or in part, for commercial purposes requires explicit permission of the authors and explicit acknowledgment of the source material (HMDB) and the original publication (see the HMDB citing page). We ask that users who download significant portions of the database cite the HMDB paper in any resulting publications. | |
Retrosynthesis Analysis
AI-Powered Synthesis Planning: Our tool employs the Template_relevance Pistachio, Template_relevance Bkms_metabolic, Template_relevance Pistachio_ringbreaker, Template_relevance Reaxys, Template_relevance Reaxys_biocatalysis model, leveraging a vast database of chemical reactions to predict feasible synthetic routes.
One-Step Synthesis Focus: Specifically designed for one-step synthesis, it provides concise and direct routes for your target compounds, streamlining the synthesis process.
Accurate Predictions: Utilizing the extensive PISTACHIO, BKMS_METABOLIC, PISTACHIO_RINGBREAKER, REAXYS, REAXYS_BIOCATALYSIS database, our tool offers high-accuracy predictions, reflecting the latest in chemical research and data.
Strategy Settings
Precursor scoring | Relevance Heuristic |
---|---|
Min. plausibility | 0.01 |
Model | Template_relevance |
Template Set | Pistachio/Bkms_metabolic/Pistachio_ringbreaker/Reaxys/Reaxys_biocatalysis |
Top-N result to add to graph | 6 |
Feasible Synthetic Routes
Q & A
Q1: What is the primary biosynthetic origin of tropic acid in plants like Datura stramonium?
A1: Research indicates that phenylalanine is the primary precursor for tropic acid biosynthesis in Datura stramonium. [, , , ] Feeding experiments with radiolabeled phenylalanine have demonstrated its incorporation into the tropic acid moiety of hyoscyamine and scopolamine. [, , , ] While tryptophan was initially proposed as a potential precursor, studies have shown its contribution to be minor in comparison to phenylalanine. []
Q2: Can you elaborate on the role of phenylalanine and other potential intermediates in the tropic acid biosynthetic pathway?
A2: While phenylalanine is established as the primary precursor, the exact sequence of intermediates leading to tropic acid is still under investigation. [, , , , ] Feeding experiments with labeled phenylpyruvate and phenyllactate suggest that phenyllactate is a closer precursor to tropic acid than phenylpyruvate. [] The stereochemistry of the conversion from phenylalanine to tropic acid has also been investigated, showing that the carboxyl group of phenylalanine migrates to its prochiral C-3 position with retention of configuration. [] Furthermore, research suggests that a mutase enzyme plays a key role in the biosynthesis of tropic acid by catalyzing the stereospecific rearrangement of 3-phenyllactate to tropate. []
Q3: How is tropic acid metabolized in different species?
A3: Metabolism of tropic acid, particularly when it is a component of larger molecules like atropine, shows significant species specificity. [] For example, in a study with Pseudomonas AT3, tropic acid was preferentially metabolized before tropine during the diauxic growth on atropine. [] In mammals, studies have shown that tropic acid is a major metabolite of scopolamine in rabbits and guinea pigs, while its significance is lower in mice and rats. []
Q4: What is the chemical structure of tropic acid?
A4: Tropic acid is an aromatic carboxylic acid with the systematic name 2-phenyl-3-hydroxypropanoic acid. Its structure consists of a benzene ring attached to a propanoic acid chain with a hydroxyl group at the β-carbon.
Q5: What are the molecular formula, weight, and key spectroscopic features of tropic acid?
A5: The molecular formula of tropic acid is C9H10O3, and its molecular weight is 166.17 g/mol. While the provided research papers do not contain detailed spectroscopic data on tropic acid itself, techniques like nuclear magnetic resonance (NMR) spectroscopy and mass spectrometry (MS) have been used to elucidate the structures of tropic acid derivatives and metabolites. [, , , , ] For instance, 13C NMR spectroscopy was instrumental in analyzing the incorporation of labeled precursors into the tropic acid moiety of hyoscyamine and understanding the stereochemical course of the mutase involved in its biosynthesis. [, ]
Q6: How does the stereochemistry of tropic acid affect its biological activity?
A6: Tropic acid possesses a chiral center, resulting in two enantiomers: (R)-(+)-tropic acid and (S)-(−)-tropic acid. The naturally occurring form, (S)-(−)-tropic acid, is the pharmacologically active enantiomer and constitutes the tropic acid moiety in hyoscyamine and scopolamine. [, , , ]
Q7: What are some notable chemical reactions involving tropic acid and its derivatives?
A7: Several studies have explored the chemical synthesis and modification of tropic acid and its derivatives. One study focused on the synthesis of tropic acid from methyl phenylacetate and paraformaldehyde. [] Other research highlighted the synthesis of tropine-labeled atropine, which involved the esterification of tropine with tropic acid. [] Furthermore, the preparation of enantiomerically pure tropic acid esters with amino alcohols has been investigated. [] These studies provide valuable insights into the synthetic manipulation of tropic acid for various applications.
Q8: What are the potential areas for future research on tropic acid?
A9: Despite extensive research, some aspects of tropic acid biosynthesis remain elusive. Investigating the intricate enzymatic machinery responsible for the rearrangement of phenylalanine to tropic acid, including the mutase enzyme, could offer valuable insights. [] Additionally, exploring the potential of metabolic engineering to enhance tropic acid production in plants or microbial systems could be of significant interest for increasing the yield of valuable tropane alkaloids.
Avertissement et informations sur les produits de recherche in vitro
Veuillez noter que tous les articles et informations sur les produits présentés sur BenchChem sont destinés uniquement à des fins informatives. Les produits disponibles à l'achat sur BenchChem sont spécifiquement conçus pour des études in vitro, qui sont réalisées en dehors des organismes vivants. Les études in vitro, dérivées du terme latin "in verre", impliquent des expériences réalisées dans des environnements de laboratoire contrôlés à l'aide de cellules ou de tissus. Il est important de noter que ces produits ne sont pas classés comme médicaments et n'ont pas reçu l'approbation de la FDA pour la prévention, le traitement ou la guérison de toute condition médicale, affection ou maladie. Nous devons souligner que toute forme d'introduction corporelle de ces produits chez les humains ou les animaux est strictement interdite par la loi. Il est essentiel de respecter ces directives pour assurer la conformité aux normes légales et éthiques en matière de recherche et d'expérimentation.