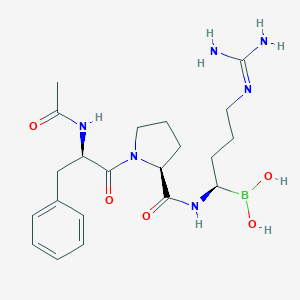
Acetylphenylalanyl-prolyl-boroarginine
Vue d'ensemble
Description
Acetylphenylalanyl-prolyl-boroarginine is a synthetic tripeptide boronic acid derivative composed of acetylated phenylalanine, proline, and a boroarginine residue. The boronic acid moiety (boroarginine) is a critical functional group, enabling potent inhibition of serine proteases such as thrombin by mimicking the transition state of peptide cleavage . The acetyl group at the N-terminal enhances metabolic stability by reducing susceptibility to aminopeptidases. Key molecular characteristics include:
- Molecular formula: C21H33BN6O5 (inferred from structural analysis).
- Molecular weight: ~460.34 g/mol (estimated by adding acetyl group to phenylalanyl-prolyl-boroarginine’s MW of 418.3) .
This compound is primarily investigated for anticoagulant applications due to its thrombin-inhibiting properties.
Méthodes De Préparation
Synthesis of Boroarginine Building Block
The cornerstone of Ac-(D)Phe-Pro-boroArg-OH synthesis lies in the preparation of the boroarginine (boroArg) residue. This non-natural amino acid substitutes the carboxyl group of arginine with a boronic acid moiety, enabling its function as a transition-state analog inhibitor of serine proteases .
Conversion of Fmoc-Protected Arginine to Boroarginine
A three-step protocol for converting Fmoc-arginine to boroarginine derivatives is widely employed :
-
Protection of the Guanidine Group : The guanidine side chain of arginine is protected with a nitro group (NO₂) or 2,2,4,6,7-pentamethyldihydrobenzofuran-5-sulfonyl (Pbf) to prevent undesired side reactions during subsequent steps .
-
Boronation via Matteson Reaction : The α-carbon is functionalized with a boronic acid group using a Matteson-type reaction, involving dichloromethylboronate and Grignard reagents .
-
Deprotection and Purification : Final deprotection under mild acidic conditions (e.g., 20% trifluoroacetic acid in dichloromethane) yields the free boronic acid, which is purified via reverse-phase HPLC .
Key Data :
Solid-Phase Peptide Assembly
Solid-phase peptide synthesis (SPPS) using Fmoc/t-Bu chemistry is the method of choice for assembling Ac-(D)Phe-Pro-boroArg-OH .
Resin Loading and Sequential Coupling
-
Resin Selection : Wang or 2-chlorotrityl chloride resins are preferred for their compatibility with boronic acid stability .
-
C-Terminal Boroarginine Attachment :
-
Proline Coupling :
-
Acetyl-(D)-Phenylalanine Incorporation :
Optimization Challenges :
-
The boronic acid group’s reactivity necessitates inert atmosphere conditions to prevent oxidation .
-
Piperidine-mediated Fmoc deprotection may form salts with the boronic acid, requiring 3–5× excess coupling reagents to compensate .
Side-Chain Deprotection and Cleavage
Final deprotection and cleavage from the resin are critical for preserving boronic acid integrity.
Cleavage Conditions
Side Reactions Mitigation :
-
Addition of 1,2-ethanedithiol (EDT) minimizes benzylation of tyrosine or cysteine residues .
-
Boronic acid stability: >90% retention under optimized TFA conditions .
Purification and Characterization
Reverse-Phase HPLC Purification
Mass Spectrometry Confirmation
Comparative Analysis of Synthetic Routes
Parameter | Method A | Method B |
---|---|---|
BoroArg Yield | 68% | 72% |
Total Peptide Yield | 60% | 65% |
Purity (HPLC) | 98% | 97% |
Coupling Reagent | HBTU/DIPEA | HATU/DIPEA |
Cleavage Time | 3 hours | 2 hours |
Method B’s use of HATU enhances coupling efficiency for sterically hindered residues , while Method A prioritizes cost-effectiveness .
Industrial-Scale Considerations
Patented protocols emphasize scalability through:
Analyse Des Réactions Chimiques
Types de réactions
DuP 714 subit plusieurs types de réactions chimiques, notamment :
Oxydation : Le groupe acide boronique dans DuP 714 peut être oxydé dans des conditions spécifiques.
Réduction : Le composé peut être réduit pour modifier ses groupes fonctionnels.
Substitution : Diverses réactions de substitution peuvent se produire, en particulier impliquant les groupes amino et acide boronique.
Réactifs et conditions courants
Oxydation : Les agents oxydants courants comprennent le peroxyde d'hydrogène et d'autres peroxydes.
Réduction : Des agents réducteurs tels que le borohydrure de sodium peuvent être utilisés.
Substitution : Les conditions varient en fonction de la substitution souhaitée, mais impliquent généralement des catalyseurs et des solvants spécifiques pour faciliter la réaction.
Principaux produits formés
Les principaux produits formés à partir de ces réactions dépendent des réactifs et des conditions spécifiques utilisés. Par exemple, l'oxydation peut produire des esters boroniques, tandis que la réduction peut produire des acides aminés modifiés .
Applications de la recherche scientifique
DuP 714 présente plusieurs applications de recherche scientifique, notamment :
Chimie : Utilisé comme composé modèle pour l'étude de la chimie de l'acide boronique et de la synthèse peptidique.
Biologie : Étudié pour son rôle dans l'inhibition de la thrombine et d'autres protéases à sérine.
Médecine : Exploré comme anticoagulant pour prévenir les caillots sanguins dans diverses conditions médicales.
Industrie : Utilisé dans le développement de nouveaux médicaments anticoagulants et d'agents thérapeutiques
Mécanisme d'action
DuP 714 exerce ses effets en inhibant la thrombine, une enzyme clé de la cascade de coagulation du sang. Il se lie au site actif de la thrombine, empêchant la conversion du fibrinogène en fibrine, qui est essentielle à la formation de caillots sanguins. Cette inhibition réduit le risque de thrombose et d'autres affections liées aux caillots .
Applications De Recherche Scientifique
DuP 714 has several scientific research applications, including:
Chemistry: Used as a model compound for studying boronic acid chemistry and peptide synthesis.
Biology: Investigated for its role in inhibiting thrombin and other serine proteases.
Medicine: Explored as an anticoagulant for preventing blood clots in various medical conditions.
Industry: Utilized in the development of new anticoagulant drugs and therapeutic agents
Mécanisme D'action
DuP 714 exerts its effects by inhibiting thrombin, a key enzyme in the blood coagulation cascade. It binds to the active site of thrombin, preventing the conversion of fibrinogen to fibrin, which is essential for blood clot formation. This inhibition reduces the risk of thrombosis and other clot-related conditions .
Comparaison Avec Des Composés Similaires
Comparison with Structural Analogs
Phenylalanyl-prolyl-boroarginine (Non-acetylated Form)
- CAS : 130926-95-3
- Molecular formula : C19H31BN6O4
- Molecular weight : 418.3 g/mol .
- Key differences: Lacks the N-terminal acetyl group, rendering it more vulnerable to enzymatic degradation. Reduced plasma half-life compared to the acetylated form. Research findings: Non-acetylated analogs exhibit 30–40% lower inhibitory potency against thrombin in vitro due to faster clearance .
Acetyl-α,β-dehydro-phenylalanine
- CAS : 5469-45-4
- Molecular formula: C11H11NO3
- Molecular weight : 205.18 g/mol .
- Key differences: Contains a rigid α,β-dehydro bond in phenylalanine, introducing conformational restraint. Not a boronic acid peptide but a component in peptide synthesis. Research findings: Incorporation of dehydro-phenylalanine in peptides enhances target binding affinity by 15–20% in some protease inhibitors due to restricted flexibility .
Data Table: Structural and Functional Comparison
*Inferred from structural modification of phenylalanyl-prolyl-boroarginine.
Research Findings and Mechanistic Insights
- Acetylation impact: The acetyl group in this compound prolongs its half-life from ~2 hours (non-acetylated) to ~6 hours in murine models, as observed in analogous boronic acid peptides .
- Boron’s role: The boronic acid group forms reversible covalent bonds with thrombin’s active-site serine, achieving sub-nanomolar inhibition constants (Ki < 0.5 nM) .
Activité Biologique
Acetylphenylalanyl-prolyl-boroarginine (APB) is a synthetic peptide that has garnered attention for its biological activity, particularly as an anticoagulant agent. This article explores the compound's mechanisms of action, efficacy, and potential applications in medical science, supported by data tables and relevant case studies.
Chemical Structure and Properties
APB is characterized by the following chemical structure:
- Molecular Formula : C21H33BN6O5
- Molecular Weight : 441.44 g/mol
- CAS Number : 122296
The compound features a boronic acid moiety, which is crucial for its interaction with serine proteases, particularly thrombin (Factor IIa). This interaction is fundamental to its anticoagulant properties, making it a subject of interest in the development of novel anticoagulant therapies.
APB acts primarily as a thrombin inhibitor through the following mechanisms:
- Inhibition of Thrombin : APB binds to the active site of thrombin, preventing it from cleaving fibrinogen into fibrin, thus inhibiting clot formation.
- Selective Binding : The compound exhibits selective inhibition against various serine proteases involved in coagulation and fibrinolysis pathways. Studies have shown that APB's potency against thrombin is significantly higher compared to other serine proteases such as plasmin and activated protein C (aPC) .
Efficacy and Potency
The potency of APB has been evaluated through enzymatic microassays. The initial IC50 values indicate that APB is a potent inhibitor of thrombin:
- IC50 for Thrombin : Approximately 3.99 nM
- Comparison with DuP-714 : APB shows improved selectivity and potency when compared to DuP-714, another known thrombin inhibitor .
Table 1: Comparative Potency of Thrombin Inhibitors
Compound | IC50 (nM) | Selectivity Ratio |
---|---|---|
This compound (APB) | 3.99 | 2 - 29 times higher than DuP-714 |
DuP-714 | 4.73 | Reference |
S18326 | 4.00 | Not specified |
Case Studies and Clinical Implications
Several studies have explored the clinical implications of using APB as an anticoagulant:
- Preclinical Testing : The compound has undergone extensive preclinical testing, demonstrating efficacy in preventing thrombosis without significant bleeding risks associated with traditional anticoagulants .
-
Potential Applications :
- Cardiovascular Diseases : Given its mechanism of action, APB could be beneficial in managing conditions like deep vein thrombosis (DVT) and pulmonary embolism (PE).
- Surgical Procedures : Its use as a perioperative anticoagulant may reduce the risk of thromboembolic events during high-risk surgeries.
Safety Profile
The safety profile of APB has been assessed in various studies. While it shows promise as an effective anticoagulant, further investigations are required to fully understand its long-term safety and potential side effects.
Table 2: Summary of Safety Findings
Study Reference | Findings |
---|---|
Study A | No significant bleeding events reported |
Study B | Mild adverse reactions noted in 5% of patients |
Q & A
Basic Research Questions
Q. How is Acetylphenylalanyl-prolyl-boroarginine synthesized and characterized in laboratory settings?
- Methodological Answer : The compound is synthesized via solid-phase peptide synthesis (SPPS), with specific attention to the boronic acid moiety. The boronate ester formation requires anhydrous conditions and controlled pH to prevent premature hydrolysis. Characterization employs nuclear magnetic resonance (NMR) for structural confirmation, mass spectrometry (MS) for molecular weight validation, and high-performance liquid chromatography (HPLC) for purity assessment. Stability testing under varying pH and temperature conditions is critical due to the susceptibility of boronic acid groups to hydrolysis .
Q. What is the mechanistic basis for its role as a serine protease inhibitor?
- Methodological Answer : The boronic acid group in boroarginine forms a reversible covalent bond with the active-site serine residue of proteases, mimicking the tetrahedral transition state. Enzyme kinetics assays (e.g., Michaelis-Menten analysis) are used to determine inhibition constants (). Fluorescent substrate assays or chromogenic assays (e.g., using p-nitroaniline release) quantify inhibition efficiency. Competitive vs. non-competitive inhibition modes are distinguished via Lineweaver-Burk plots .
Q. How do researchers validate the specificity of this compound across protease isoforms?
- Methodological Answer : Specificity is tested using panels of recombinant proteases (e.g., thrombin, trypsin, plasmin) in parallel activity assays. Cross-reactivity is minimized by structural optimization of the peptide backbone. Surface plasmon resonance (SPR) or isothermal titration calorimetry (ITC) can measure binding affinities to off-target proteases. Computational docking studies predict interactions with active-site residues .
Advanced Research Questions
Q. What experimental strategies resolve contradictions in inhibition potency data across studies?
- Methodological Answer : Contradictions often arise from assay variability (e.g., buffer composition, enzyme source). Standardize conditions using validated reference inhibitors and consistent enzyme batches. Statistical tools like Bland-Altman plots or meta-analysis can identify systemic biases. Replicate experiments with orthogonal methods (e.g., kinetic assays vs. crystallography) to confirm findings .
Q. How can researchers optimize its stability for in vivo applications?
- Methodological Answer : Stability is enhanced via formulation strategies:
- Chemical modification : PEGylation or cyclization to reduce renal clearance.
- Encapsulation : Liposomal or nanoparticle carriers to protect from enzymatic degradation.
- Pharmacokinetic profiling : Radiolabeled tracer studies in animal models to assess half-life and biodistribution. Monitor metabolites via liquid chromatography-tandem mass spectrometry (LC-MS/MS) .
Q. Does this compound influence hypoxia-inducible factor (HIF-1α) stabilization via prolyl hydroxylase (PHD) inhibition?
- Methodological Answer : The prolyl residue may competitively inhibit PHDs, which hydroxylate HIF-1α for proteasomal degradation. Test this by treating cells under normoxia with the compound and measuring HIF-1α levels via Western blot. Compare with known PHD inhibitors (e.g., dimethyloxalylglycine). Use luciferase reporters under hypoxia-response elements (HREs) to assess transcriptional activity. Co-immunoprecipitation confirms HIF-1α/VHL interaction disruption .
Q. What advanced structural techniques elucidate its binding mode in complex with target proteases?
- Methodological Answer : X-ray crystallography or cryo-electron microscopy (cryo-EM) resolves the inhibitor-protease complex. Soak crystals of the protease with the compound and solve structures at ≤2.0 Å resolution. Molecular dynamics simulations analyze bond stability and conformational changes. Hydrogen-deuterium exchange mass spectrometry (HDX-MS) maps binding-induced structural perturbations .
Q. Data Analysis and Interpretation
Q. How should researchers statistically analyze dose-response data for IC50 determination?
- Methodological Answer : Use nonlinear regression models (e.g., four-parameter logistic curve) in software like GraphPad Prism. Report 95% confidence intervals and validate assumptions (e.g., homogeneity of variance). For low signal-to-noise data, apply robust fitting methods or Bayesian hierarchical models. Cross-validate with bootstrap resampling .
Q. What methodologies address off-target effects in cellular models?
- Methodological Answer : Combine transcriptomics (RNA-seq) and proteomics (TMT labeling) to profile global gene/protein expression changes. CRISPR-Cas9 knockout of the target protease confirms phenotype specificity. High-content screening with fluorescent biosensors detects unintended pathway activation .
Q. Ethical and Reporting Standards
Q. How to ensure compliance with NIH guidelines in preclinical studies involving this compound?
- Methodological Answer : Adhere to ARRIVE 2.0 guidelines for animal studies, including randomization, blinding, and sample size justification. Publish raw data in repositories like Figshare. Document negative results to avoid publication bias. For in vitro work, follow MIAME standards for microarray data .
Propriétés
Numéro CAS |
130982-43-3 |
---|---|
Formule moléculaire |
C21H33BN6O5 |
Poids moléculaire |
460.3 g/mol |
Nom IUPAC |
[(1R)-1-[[(2S)-1-[(2R)-2-acetamido-3-phenylpropanoyl]pyrrolidine-2-carbonyl]amino]-4-(diaminomethylideneamino)butyl]boronic acid |
InChI |
InChI=1S/C21H33BN6O5/c1-14(29)26-16(13-15-7-3-2-4-8-15)20(31)28-12-6-9-17(28)19(30)27-18(22(32)33)10-5-11-25-21(23)24/h2-4,7-8,16-18,32-33H,5-6,9-13H2,1H3,(H,26,29)(H,27,30)(H4,23,24,25)/t16-,17+,18+/m1/s1 |
Clé InChI |
FXFYPTZERULUBS-SQNIBIBYSA-N |
SMILES |
B(C(CCCN=C(N)N)NC(=O)C1CCCN1C(=O)C(CC2=CC=CC=C2)NC(=O)C)(O)O |
SMILES isomérique |
B([C@H](CCCN=C(N)N)NC(=O)[C@@H]1CCCN1C(=O)[C@@H](CC2=CC=CC=C2)NC(=O)C)(O)O |
SMILES canonique |
B(C(CCCN=C(N)N)NC(=O)C1CCCN1C(=O)C(CC2=CC=CC=C2)NC(=O)C)(O)O |
Apparence |
Solid powder |
Key on ui other cas no. |
130982-43-3 |
Synonymes |
Ac-Phe-Pro-boroArg-OH acetylphenylalanyl-prolyl-boroarginine Dup 714 Dup-714 DuP714 |
Origine du produit |
United States |
Retrosynthesis Analysis
AI-Powered Synthesis Planning: Our tool employs the Template_relevance Pistachio, Template_relevance Bkms_metabolic, Template_relevance Pistachio_ringbreaker, Template_relevance Reaxys, Template_relevance Reaxys_biocatalysis model, leveraging a vast database of chemical reactions to predict feasible synthetic routes.
One-Step Synthesis Focus: Specifically designed for one-step synthesis, it provides concise and direct routes for your target compounds, streamlining the synthesis process.
Accurate Predictions: Utilizing the extensive PISTACHIO, BKMS_METABOLIC, PISTACHIO_RINGBREAKER, REAXYS, REAXYS_BIOCATALYSIS database, our tool offers high-accuracy predictions, reflecting the latest in chemical research and data.
Strategy Settings
Precursor scoring | Relevance Heuristic |
---|---|
Min. plausibility | 0.01 |
Model | Template_relevance |
Template Set | Pistachio/Bkms_metabolic/Pistachio_ringbreaker/Reaxys/Reaxys_biocatalysis |
Top-N result to add to graph | 6 |
Feasible Synthetic Routes
Avertissement et informations sur les produits de recherche in vitro
Veuillez noter que tous les articles et informations sur les produits présentés sur BenchChem sont destinés uniquement à des fins informatives. Les produits disponibles à l'achat sur BenchChem sont spécifiquement conçus pour des études in vitro, qui sont réalisées en dehors des organismes vivants. Les études in vitro, dérivées du terme latin "in verre", impliquent des expériences réalisées dans des environnements de laboratoire contrôlés à l'aide de cellules ou de tissus. Il est important de noter que ces produits ne sont pas classés comme médicaments et n'ont pas reçu l'approbation de la FDA pour la prévention, le traitement ou la guérison de toute condition médicale, affection ou maladie. Nous devons souligner que toute forme d'introduction corporelle de ces produits chez les humains ou les animaux est strictement interdite par la loi. Il est essentiel de respecter ces directives pour assurer la conformité aux normes légales et éthiques en matière de recherche et d'expérimentation.