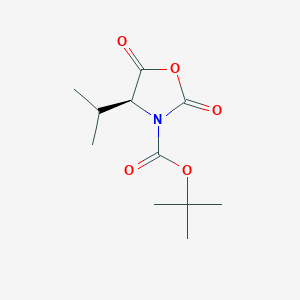
N-carboxyanhydride de Boc-L-valine
Vue d'ensemble
Description
Boc-L-Valine N-carboxyanhydride is a useful research compound. Its molecular formula is C11H17NO5 and its molecular weight is 243.26 g/mol. The purity is usually 95%.
BenchChem offers high-quality Boc-L-Valine N-carboxyanhydride suitable for many research applications. Different packaging options are available to accommodate customers' requirements. Please inquire for more information about Boc-L-Valine N-carboxyanhydride including the price, delivery time, and more detailed information at info@benchchem.com.
Applications De Recherche Scientifique
Peptides synthétiques en cosmétique
Le N-carboxyanhydride de Boc-L-valine est utilisé dans la synthèse de peptides, qui sont utilisés en cosmétique pour les peaux sensibles . Ces peptides interagissent avec les cellules de la peau par le biais de plusieurs mécanismes et ont une forte puissance à faibles doses . Ils sont capables de pénétrer la couche cornée, la couche la plus externe de la peau .
Synthèse d'alcaloïdes thérapeutiques
Ce composé peut être préparé directement à partir d'acides aminés et de CO2 en utilisant de l'anhydride n-propylphosphonique . Les N-carboxyanhydrides (NCA) qui en résultent peuvent être transformés en alcaloïdes à activité médicinale tels que la tryptanthrine et la phaitanthrine A en un seul pot .
Monomère de protection des acides aminés
La N-Boc-L-valine est utilisée comme monomère de protection des acides aminés dans la synthèse de plusieurs peptides . Ce processus est crucial dans la production de peptides complexes, qui ont de nombreuses applications dans la recherche biologique et le développement de médicaments .
Activité antimicrobienne
Le composé a été utilisé dans la synthèse de polypeptides en forme d'étoile, qui ont montré une activité antimicrobienne accrue . Ces polymères détruisent les membranes des microbes, ce qui en fait un outil potentiel dans la lutte contre les infections .
Transformations chimiques
Le groupe tert-butyle du composé présente des schémas de réactivité uniques, qui sont utiles dans diverses transformations chimiques
Mécanisme D'action
Target of Action
Boc-L-Valine N-carboxyanhydride, also known as tert-butyl (4S)-2,5-dioxo-4-propan-2-yl-1,3-oxazolidine-3-carboxylate, is a derivative of amino acids . The primary targets of this compound are proteins and peptides in the body. It interacts with these targets to initiate a series of biochemical reactions .
Mode of Action
The compound acts as a building block in the synthesis of polypeptides . It undergoes ring-opening polymerization to form homopolypeptides . This process is catalyzed by metal catalysts . The resulting polypeptides can then interact with various biological targets to exert their effects .
Biochemical Pathways
The key biochemical pathway involved in the action of Boc-L-Valine N-carboxyanhydride is the formation of polypeptides through ring-opening polymerization . This process is crucial for the synthesis of proteins and peptides, which play vital roles in numerous biological processes .
Pharmacokinetics
Given its role in the synthesis of polypeptides, it is likely that its absorption, distribution, metabolism, and excretion (adme) properties would be influenced by factors such as the presence of appropriate catalysts and the physiological environment .
Result of Action
The primary result of the action of Boc-L-Valine N-carboxyanhydride is the formation of polypeptides . These polypeptides can then participate in various biological processes, potentially influencing cellular functions and contributing to the overall physiological response .
Action Environment
The action of Boc-L-Valine N-carboxyanhydride is influenced by various environmental factors. For instance, the presence of appropriate catalysts is crucial for its ring-opening polymerization . Additionally, factors such as pH and temperature could potentially influence its stability and efficacy .
Activité Biologique
Boc-L-Valine N-carboxyanhydride (Boc-L-Val-NCA) is a cyclic derivative of the amino acid valine, commonly used in peptide synthesis and polymer chemistry. This compound has garnered attention for its potential biological activities, particularly in antimicrobial applications and its role in the synthesis of polypeptides. This article explores the biological activity of Boc-L-Val-NCA, highlighting relevant research findings, data tables, and case studies.
Overview of Boc-L-Valine N-Carboxyanhydride
- Chemical Structure : Boc-L-Val-NCA is derived from L-valine with a tert-butyloxycarbonyl (Boc) protecting group on the amino group. The N-carboxyanhydride form allows for ring-opening polymerization (ROP), facilitating the synthesis of polypeptides.
- Molecular Formula : CHNO
- Molecular Weight : 217.26 g/mol
Antimicrobial Properties
Research indicates that compounds derived from valine, including Boc-L-Val-NCA, exhibit significant antimicrobial properties. A study focusing on derivatives of moiramide B, which incorporates valine units, demonstrated potent activity against various bacterial strains including Staphylococcus aureus and Bacillus subtilis, with minimum inhibitory concentrations (MICs) as low as 1 μg/mL .
Compound | MIC against S. aureus (μg/mL) | MIC against B. subtilis (μg/mL) |
---|---|---|
Moiramide B Derivative 1 | 1 | 2 |
Moiramide B Derivative 2 | 0.5 | 3 |
Boc-L-Val-NCA | TBD | TBD |
Note: TBD indicates that specific MIC values for Boc-L-Val-NCA were not provided in the reviewed literature.
The mechanism by which Boc-L-Val-NCA exerts its biological effects is primarily associated with its ability to interfere with bacterial cell wall synthesis. It acts as an inhibitor of acetyl-CoA carboxylase (ACC), a key enzyme in fatty acid biosynthesis . This inhibition disrupts the assembly of bacterial cell walls, leading to cell lysis and death.
Synthesis and Evaluation of Peptide Analogues
In a study examining the synthesis of antimicrobial peptides using Boc-L-Val-NCA as a building block, researchers synthesized several peptide analogues to evaluate their biological activities. These analogues were designed to mimic natural antimicrobial peptides (AMPs) while incorporating non-proteinogenic amino acids to enhance selectivity and potency against pathogens.
- Findings : The modified peptides demonstrated enhanced antimicrobial activity compared to their natural counterparts, with some variants showing effective bacteriolysis against resistant strains of Escherichia coli and Staphylococcus aureus .
Polymerization Studies
The ring-opening polymerization of Boc-L-Val-NCA has been extensively studied for its application in creating biocompatible polymers for drug delivery systems. Research has shown that polymers derived from Boc-L-Val-NCA can be tailored to improve drug solubility and release profiles .
- Research Outcome : Polymers synthesized from Boc-L-Val-NCA exhibited lower cytotoxicity levels while maintaining effective drug delivery capabilities, making them suitable candidates for further development in pharmaceutical applications.
Propriétés
IUPAC Name |
tert-butyl (4S)-2,5-dioxo-4-propan-2-yl-1,3-oxazolidine-3-carboxylate | |
---|---|---|
Source | PubChem | |
URL | https://pubchem.ncbi.nlm.nih.gov | |
Description | Data deposited in or computed by PubChem | |
InChI |
InChI=1S/C11H17NO5/c1-6(2)7-8(13)16-9(14)12(7)10(15)17-11(3,4)5/h6-7H,1-5H3/t7-/m0/s1 | |
Source | PubChem | |
URL | https://pubchem.ncbi.nlm.nih.gov | |
Description | Data deposited in or computed by PubChem | |
InChI Key |
RTIIPUBJZYTAMU-ZETCQYMHSA-N | |
Source | PubChem | |
URL | https://pubchem.ncbi.nlm.nih.gov | |
Description | Data deposited in or computed by PubChem | |
Canonical SMILES |
CC(C)C1C(=O)OC(=O)N1C(=O)OC(C)(C)C | |
Source | PubChem | |
URL | https://pubchem.ncbi.nlm.nih.gov | |
Description | Data deposited in or computed by PubChem | |
Isomeric SMILES |
CC(C)[C@H]1C(=O)OC(=O)N1C(=O)OC(C)(C)C | |
Source | PubChem | |
URL | https://pubchem.ncbi.nlm.nih.gov | |
Description | Data deposited in or computed by PubChem | |
Molecular Formula |
C11H17NO5 | |
Source | PubChem | |
URL | https://pubchem.ncbi.nlm.nih.gov | |
Description | Data deposited in or computed by PubChem | |
DSSTOX Substance ID |
DTXSID801165275 | |
Record name | tert-Butyl (4S)-4-isopropyl-2,5-dioxo-1,3-oxazolidin-3-carboxylate | |
Source | EPA DSSTox | |
URL | https://comptox.epa.gov/dashboard/DTXSID801165275 | |
Description | DSSTox provides a high quality public chemistry resource for supporting improved predictive toxicology. | |
Molecular Weight |
243.26 g/mol | |
Source | PubChem | |
URL | https://pubchem.ncbi.nlm.nih.gov | |
Description | Data deposited in or computed by PubChem | |
CAS No. |
141468-55-5 | |
Record name | tert-Butyl (4S)-4-isopropyl-2,5-dioxo-1,3-oxazolidin-3-carboxylate | |
Source | CAS Common Chemistry | |
URL | https://commonchemistry.cas.org/detail?cas_rn=141468-55-5 | |
Description | CAS Common Chemistry is an open community resource for accessing chemical information. Nearly 500,000 chemical substances from CAS REGISTRY cover areas of community interest, including common and frequently regulated chemicals, and those relevant to high school and undergraduate chemistry classes. This chemical information, curated by our expert scientists, is provided in alignment with our mission as a division of the American Chemical Society. | |
Explanation | The data from CAS Common Chemistry is provided under a CC-BY-NC 4.0 license, unless otherwise stated. | |
Record name | tert-Butyl (4S)-4-isopropyl-2,5-dioxo-1,3-oxazolidin-3-carboxylate | |
Source | EPA DSSTox | |
URL | https://comptox.epa.gov/dashboard/DTXSID801165275 | |
Description | DSSTox provides a high quality public chemistry resource for supporting improved predictive toxicology. | |
Avertissement et informations sur les produits de recherche in vitro
Veuillez noter que tous les articles et informations sur les produits présentés sur BenchChem sont destinés uniquement à des fins informatives. Les produits disponibles à l'achat sur BenchChem sont spécifiquement conçus pour des études in vitro, qui sont réalisées en dehors des organismes vivants. Les études in vitro, dérivées du terme latin "in verre", impliquent des expériences réalisées dans des environnements de laboratoire contrôlés à l'aide de cellules ou de tissus. Il est important de noter que ces produits ne sont pas classés comme médicaments et n'ont pas reçu l'approbation de la FDA pour la prévention, le traitement ou la guérison de toute condition médicale, affection ou maladie. Nous devons souligner que toute forme d'introduction corporelle de ces produits chez les humains ou les animaux est strictement interdite par la loi. Il est essentiel de respecter ces directives pour assurer la conformité aux normes légales et éthiques en matière de recherche et d'expérimentation.