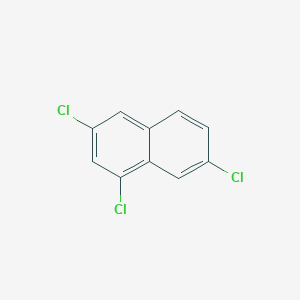
1,3,7-Trichloronaphthalene
Vue d'ensemble
Description
1,3,7-Trichloronaphthalene, also known as this compound, is a useful research compound. Its molecular formula is C10H5Cl3 and its molecular weight is 231.5 g/mol. The purity is usually 95%.
The exact mass of the compound this compound is unknown and the complexity rating of the compound is unknown. The solubility of this chemical has been described as 2.78e-07 m. The storage condition is unknown. Please store according to label instructions upon receipt of goods.
BenchChem offers high-quality this compound suitable for many research applications. Different packaging options are available to accommodate customers' requirements. Please inquire for more information about this compound including the price, delivery time, and more detailed information at info@benchchem.com.
Applications De Recherche Scientifique
Calcul du facteur de bioconcentration
Le 1,3,7-trichloronaphtalène est utilisé dans la recherche pour calculer le facteur de bioconcentration (BCF). Cette méthode validée pour l'analyse du 1,3,7-trichloronaphtalène est basée sur une méthode expérimentale validée. Elle utilise un ensemble de coefficients structuraux et une valeur expérimentale pour calculer le BCF . Le BCF est ensuite utilisé pour calculer le vecteur optimal, qui est trouvé en utilisant des descripteurs et des facteurs structuraux .
Liaison au récepteur des hydrocarbures aromatiques
Le 1,3,7-trichloronaphtalène est un chloronaphtalène (CN) qui peut se lier au récepteur des hydrocarbures aromatiques (AhR) . L'AhR est une protéine qui régule l'expression de plusieurs gènes impliqués dans la réponse immunitaire et la régulation du cycle cellulaire. Par conséquent, la compréhension de la liaison du 1,3,7-trichloronaphtalène à l'AhR peut fournir des informations sur ces processus biologiques .
Étalons analytiques
Le 1,3,7-trichloronaphtalène est utilisé comme étalon analytique dans la recherche en neurologie . Les étalons analytiques sont des substances avec une concentration connue d'un certain composé. Ils sont utilisés en laboratoire pour garantir la précision des dosages et des tests .
Mécanisme D'action
Target of Action
1,3,7-Trichloronaphthalene is a chloronaphthalene (CN) that can bind to the aryl hydrocarbon receptor (AhR) . The AhR is a type of protein that regulates the expression of several genes involved in biological processes such as cell growth and response to environmental changes.
Mode of Action
The interaction of this compound with its target, the AhR, leads to changes in the receptor’s activity. This interaction can alter the expression of genes regulated by the AhR, leading to various cellular responses . .
Pharmacokinetics
Based on its chemical properties, it can be inferred that it is likely to have low water solubility and high lipid solubility, which could influence its absorption and distribution in the body
Result of Action
Given its interaction with the AhR, it is likely to influence gene expression and cellular responses regulated by this receptor
Action Environment
Environmental factors can influence the action, efficacy, and stability of this compound. For instance, exposure to light can lead to its photodegradation Additionally, its stability and efficacy can be influenced by factors such as temperature, pH, and the presence of other chemicals in the environment
Propriétés
IUPAC Name |
1,3,7-trichloronaphthalene | |
---|---|---|
Source | PubChem | |
URL | https://pubchem.ncbi.nlm.nih.gov | |
Description | Data deposited in or computed by PubChem | |
InChI |
InChI=1S/C10H5Cl3/c11-7-2-1-6-3-8(12)5-10(13)9(6)4-7/h1-5H | |
Source | PubChem | |
URL | https://pubchem.ncbi.nlm.nih.gov | |
Description | Data deposited in or computed by PubChem | |
InChI Key |
CFEUGIGSIREATC-UHFFFAOYSA-N | |
Source | PubChem | |
URL | https://pubchem.ncbi.nlm.nih.gov | |
Description | Data deposited in or computed by PubChem | |
Canonical SMILES |
C1=CC(=CC2=C(C=C(C=C21)Cl)Cl)Cl | |
Source | PubChem | |
URL | https://pubchem.ncbi.nlm.nih.gov | |
Description | Data deposited in or computed by PubChem | |
Molecular Formula |
C10H5Cl3 | |
Source | PubChem | |
URL | https://pubchem.ncbi.nlm.nih.gov | |
Description | Data deposited in or computed by PubChem | |
DSSTOX Substance ID |
DTXSID00204263 | |
Record name | 1,3,7-Trichloronaphthalene | |
Source | EPA DSSTox | |
URL | https://comptox.epa.gov/dashboard/DTXSID00204263 | |
Description | DSSTox provides a high quality public chemistry resource for supporting improved predictive toxicology. | |
Molecular Weight |
231.5 g/mol | |
Source | PubChem | |
URL | https://pubchem.ncbi.nlm.nih.gov | |
Description | Data deposited in or computed by PubChem | |
CAS No. |
55720-37-1 | |
Record name | Naphthalene, 1,3,7-trichloro- | |
Source | ChemIDplus | |
URL | https://pubchem.ncbi.nlm.nih.gov/substance/?source=chemidplus&sourceid=0055720371 | |
Description | ChemIDplus is a free, web search system that provides access to the structure and nomenclature authority files used for the identification of chemical substances cited in National Library of Medicine (NLM) databases, including the TOXNET system. | |
Record name | 1,3,7-Trichloronaphthalene | |
Source | EPA DSSTox | |
URL | https://comptox.epa.gov/dashboard/DTXSID00204263 | |
Description | DSSTox provides a high quality public chemistry resource for supporting improved predictive toxicology. | |
Retrosynthesis Analysis
AI-Powered Synthesis Planning: Our tool employs the Template_relevance Pistachio, Template_relevance Bkms_metabolic, Template_relevance Pistachio_ringbreaker, Template_relevance Reaxys, Template_relevance Reaxys_biocatalysis model, leveraging a vast database of chemical reactions to predict feasible synthetic routes.
One-Step Synthesis Focus: Specifically designed for one-step synthesis, it provides concise and direct routes for your target compounds, streamlining the synthesis process.
Accurate Predictions: Utilizing the extensive PISTACHIO, BKMS_METABOLIC, PISTACHIO_RINGBREAKER, REAXYS, REAXYS_BIOCATALYSIS database, our tool offers high-accuracy predictions, reflecting the latest in chemical research and data.
Strategy Settings
Precursor scoring | Relevance Heuristic |
---|---|
Min. plausibility | 0.01 |
Model | Template_relevance |
Template Set | Pistachio/Bkms_metabolic/Pistachio_ringbreaker/Reaxys/Reaxys_biocatalysis |
Top-N result to add to graph | 6 |
Feasible Synthetic Routes
Avertissement et informations sur les produits de recherche in vitro
Veuillez noter que tous les articles et informations sur les produits présentés sur BenchChem sont destinés uniquement à des fins informatives. Les produits disponibles à l'achat sur BenchChem sont spécifiquement conçus pour des études in vitro, qui sont réalisées en dehors des organismes vivants. Les études in vitro, dérivées du terme latin "in verre", impliquent des expériences réalisées dans des environnements de laboratoire contrôlés à l'aide de cellules ou de tissus. Il est important de noter que ces produits ne sont pas classés comme médicaments et n'ont pas reçu l'approbation de la FDA pour la prévention, le traitement ou la guérison de toute condition médicale, affection ou maladie. Nous devons souligner que toute forme d'introduction corporelle de ces produits chez les humains ou les animaux est strictement interdite par la loi. Il est essentiel de respecter ces directives pour assurer la conformité aux normes légales et éthiques en matière de recherche et d'expérimentation.