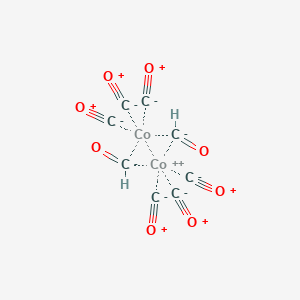
Cobalt, di-mu-carbonylhexacarbonyldi-, (Co-Co)
- Cliquez sur DEMANDE RAPIDE pour recevoir un devis de notre équipe d'experts.
- Avec des produits de qualité à un prix COMPÉTITIF, vous pouvez vous concentrer davantage sur votre recherche.
Vue d'ensemble
Description
Cobalt, di-mu-carbonylhexacarbonyldi-, (Co-Co) is a compound with the molecular formula C8Co2O8 . It is also known by other names such as Cobalt carbonyl, Cobalt octacarbonyl, Cobalt tetracarbonyl dimer, Dicobalt carbonyl, Dicobalt octacarbonyl, Di-mu-carbonylhexacarbonyldicobalt, and Octacarbonyldicobalt .
Synthesis Analysis
The synthesis of Cobalt, di-mu-carbonylhexacarbonyldi-, (Co-Co) involves the preparation of cobalt and rhodium carbonyls by reductive carbonylation with pentacarbonyliron .Molecular Structure Analysis
The molecular structure of Cobalt, di-mu-carbonylhexacarbonyldi-, (Co-Co) is represented by the InChI string:InChI=1S/2CHO.6CO.2Co/c8*1-2;;/h2*1H;;;;;;;;/q2*-1;;;;;;;;+2
. The Canonical SMILES representation is [CH-]=O. [CH-]=O. [C-]# [O+]. [C-]# [O+]. [C-]# [O+]. [C-]# [O+]. [C-]# [O+]. [C-]# [O+]. [Co]. [Co+2]
. Physical And Chemical Properties Analysis
The molecular weight of Cobalt, di-mu-carbonylhexacarbonyldi-, (Co-Co) is 343.96 g/mol . It has a complexity of 157 and a topological polar surface area of 40.1 Ų . The compound is not a hydrogen bond donor but is a hydrogen bond acceptor .Applications De Recherche Scientifique
Hydroformylation Catalyst
Dicobalt octacarbonyl is used as a catalyst in hydroformylation reactions, which convert alkenes into aldehydes, an important process in the production of fine chemicals and pharmaceuticals .
Alkyne Protection
In organic synthesis, it serves as an alkyne-protecting group, stabilizing strained cycloalkynes and preventing unwanted side reactions .
3. [2 + 2 + 2] Cycloadditions This compound is a key component in [2 + 2 + 2] cycloaddition reactions, which are useful for constructing complex cyclic structures .
Nicholas Reactions
It is utilized in Nicholas reactions, which are employed for the synthesis of complex organic molecules .
Pauson-Khand Reactions
Dicobalt octacarbonyl is also involved in Pauson-Khand reactions (PKRs), which are used to synthesize cyclopentenones, a core structure in many natural products .
Polymer Science Applications
The compound finds applications in polymer science, likely related to its ability to mediate polymerization reactions .
Synthesis of Cobalt Nanoparticles
It has been used for the synthesis of cobalt nanoparticles, which have potential applications in electronics and catalysis .
Safety and Hazards
Mécanisme D'action
Target of Action
The primary targets of carbon monoxide (CO) are heme-containing proteins such as cytochrome-c oxidase, cytochrome P450, and tryptophan dioxygenase . CO, though chemically inert, acts as a heme iron ligand . The binding affinity of carbon monoxide for hemoglobin is more than 200 times greater than that of oxygen for hemoglobin . Cobalt, on the other hand, has been shown to be involved in the catalytic efficiency of electrochemical carbon monoxide reduction to methanol .
Mode of Action
CO is continuously produced in mammals. The intracellular levels of CO can increase under stressful conditions following induction of HO-1 (heme oxygnase-1), a ubiquitous enzyme responsible for the catabolism of heme . Cobalt phthalocyanine, from the low-spin state (S=1/2) to the high-spin state (S=3/2), induced by molecular conformation change, is responsible for the greatly enhanced CO reduction reaction .
Biochemical Pathways
CO has a diverse array of physiological functions in plants. In response to environmental stress and under specific developmental conditions, endogenous CO production is induced . The biosynthesis, biodistribution, and physiological consequences of carbon monoxide (CO) in humans are intimately linked to the utilization of the heme molecule in vital cellular processes .
Pharmacokinetics
The main function of CO in humans is based on its role in vitamin B12 (cobalamin), the biological reservoir of CO .
Result of Action
CO has been shown to impact the regulation of multiple signaling pathways leading to altered cellular functions . It performs a crucial role in plant growth and development, beginning with germination and ending in the senescence of organs . The anti-inflammatory properties of CO and CO-releasing molecules (CO-RMs) have been demonstrated in a multitude of animal models of inflammation .
Action Environment
Environmental factors such as atmospheric CO2, temperature, precipitation, and radiation can affect the resource availability and carbon allocation . Accumulation of Co in agriculture and humans, due to natural and anthropogenic factors, represents a global problem affecting water quality and human and animal health .
Propriétés
IUPAC Name |
carbon monoxide;cobalt;cobalt(2+);methanone |
Source
|
---|---|---|
Source | PubChem | |
URL | https://pubchem.ncbi.nlm.nih.gov | |
Description | Data deposited in or computed by PubChem | |
InChI |
InChI=1S/2CHO.6CO.2Co/c8*1-2;;/h2*1H;;;;;;;;/q2*-1;;;;;;;;+2 |
Source
|
Source | PubChem | |
URL | https://pubchem.ncbi.nlm.nih.gov | |
Description | Data deposited in or computed by PubChem | |
InChI Key |
BXCQGSQPWPGFIV-UHFFFAOYSA-N |
Source
|
Source | PubChem | |
URL | https://pubchem.ncbi.nlm.nih.gov | |
Description | Data deposited in or computed by PubChem | |
Canonical SMILES |
[CH-]=O.[CH-]=O.[C-]#[O+].[C-]#[O+].[C-]#[O+].[C-]#[O+].[C-]#[O+].[C-]#[O+].[Co].[Co+2] |
Source
|
Source | PubChem | |
URL | https://pubchem.ncbi.nlm.nih.gov | |
Description | Data deposited in or computed by PubChem | |
Molecular Formula |
C8H2Co2O8 |
Source
|
Source | PubChem | |
URL | https://pubchem.ncbi.nlm.nih.gov | |
Description | Data deposited in or computed by PubChem | |
Molecular Weight |
343.96 g/mol |
Source
|
Source | PubChem | |
URL | https://pubchem.ncbi.nlm.nih.gov | |
Description | Data deposited in or computed by PubChem | |
Product Name |
Cobalt, di-mu-carbonylhexacarbonyldi-, (Co-Co) | |
CAS RN |
10210-68-1 |
Source
|
Record name | Cobalt, di-.mu.-carbonylhexacarbonyldi-, (Co-Co) | |
Source | EPA Chemicals under the TSCA | |
URL | https://www.epa.gov/chemicals-under-tsca | |
Description | EPA Chemicals under the Toxic Substances Control Act (TSCA) collection contains information on chemicals and their regulations under TSCA, including non-confidential content from the TSCA Chemical Substance Inventory and Chemical Data Reporting. | |
Avertissement et informations sur les produits de recherche in vitro
Veuillez noter que tous les articles et informations sur les produits présentés sur BenchChem sont destinés uniquement à des fins informatives. Les produits disponibles à l'achat sur BenchChem sont spécifiquement conçus pour des études in vitro, qui sont réalisées en dehors des organismes vivants. Les études in vitro, dérivées du terme latin "in verre", impliquent des expériences réalisées dans des environnements de laboratoire contrôlés à l'aide de cellules ou de tissus. Il est important de noter que ces produits ne sont pas classés comme médicaments et n'ont pas reçu l'approbation de la FDA pour la prévention, le traitement ou la guérison de toute condition médicale, affection ou maladie. Nous devons souligner que toute forme d'introduction corporelle de ces produits chez les humains ou les animaux est strictement interdite par la loi. Il est essentiel de respecter ces directives pour assurer la conformité aux normes légales et éthiques en matière de recherche et d'expérimentation.