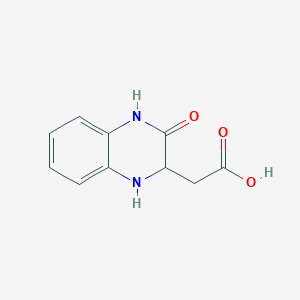
Acide 1,2,3,4-tétrahydro-3-oxo-2-quinoxalinecétique
Vue d'ensemble
Description
1,2,3,4-Tetrahydro-3-oxo-2-quinoxalineacetic acid is an organic compound with the molecular formula C10H10N2O3 and a molecular weight of 206.2 g/mol It is known for its unique structure, which includes a quinoxaline ring system fused with a tetrahydro-3-oxo moiety
Applications De Recherche Scientifique
1,2,3,4-Tetrahydro-3-oxo-2-quinoxalineacetic acid has a wide range of scientific research applications, including:
Méthodes De Préparation
Synthetic Routes and Reaction Conditions
The synthesis of 1,2,3,4-Tetrahydro-3-oxo-2-quinoxalineacetic acid typically involves the condensation of appropriate starting materials under controlled conditions. One common method involves the reaction of 2-quinoxalinecarboxylic acid with a reducing agent to form the tetrahydro derivative . The reaction conditions often include the use of solvents such as ethanol or dimethyl sulfoxide (DMSO) and may require heating to facilitate the reaction.
Industrial Production Methods
Industrial production of 1,2,3,4-Tetrahydro-3-oxo-2-quinoxalineacetic acid may involve large-scale synthesis using similar reaction conditions as in laboratory settings. The process is optimized for higher yields and purity, often employing advanced techniques such as continuous flow reactors and automated synthesis systems .
Analyse Des Réactions Chimiques
Types of Reactions
1,2,3,4-Tetrahydro-3-oxo-2-quinoxalineacetic acid undergoes various chemical reactions, including:
Oxidation: The compound can be oxidized to form quinoxaline derivatives.
Reduction: Reduction reactions can yield tetrahydroquinoxaline derivatives.
Common Reagents and Conditions
Common reagents used in these reactions include oxidizing agents such as potassium permanganate, reducing agents like sodium borohydride, and nucleophiles such as amines and thiols. Reaction conditions may vary depending on the desired product, but typically involve controlled temperatures and specific solvents .
Major Products Formed
The major products formed from these reactions include various quinoxaline derivatives, which can be further functionalized for specific applications in research and industry .
Mécanisme D'action
The mechanism of action of 1,2,3,4-Tetrahydro-3-oxo-2-quinoxalineacetic acid involves its interaction with specific molecular targets and pathways. The compound can act as an inhibitor of certain enzymes, affecting their activity and leading to various biological effects. The exact molecular targets and pathways involved are still under investigation, but studies suggest that it may interact with proteins involved in cell signaling and metabolic pathways .
Comparaison Avec Des Composés Similaires
Similar Compounds
Similar compounds to 1,2,3,4-Tetrahydro-3-oxo-2-quinoxalineacetic acid include:
- 2-Quinoxalineacetic acid
- 1,2,3,4-Tetrahydro-3-isoquinolinecarboxylic acid
- 2-Oxo-1,2,3,4-tetrahydroquinolinecarboxylic acid
Uniqueness
What sets 1,2,3,4-Tetrahydro-3-oxo-2-quinoxalineacetic acid apart from these similar compounds is its specific structure, which combines a quinoxaline ring with a tetrahydro-3-oxo moiety. This unique structure imparts distinct chemical and biological properties, making it a valuable compound for various research applications .
Activité Biologique
1,2,3,4-Tetrahydro-3-oxo-2-quinoxalineacetic acid (THQA) is a synthetic organic compound with the molecular formula C10H10N2O3 and a molecular weight of 206.2 g/mol. It belongs to the quinoxaline family, which is recognized for its diverse biological activities and potential therapeutic applications. This article explores the biological activity of THQA, focusing on its mechanisms of action, therapeutic potential, and relevant research findings.
The biological activity of THQA is primarily attributed to its interaction with various molecular targets involved in cellular signaling and metabolic pathways. Preliminary studies suggest that THQA may function as an inhibitor of specific enzymes, thereby affecting their activity and leading to various biological effects. The exact molecular targets are still under investigation but may include proteins involved in cell signaling cascades and metabolic regulation .
Antimicrobial Activity
Research has indicated that THQA exhibits significant antimicrobial properties. In vitro studies have demonstrated its effectiveness against a range of pathogenic microorganisms, including Gram-positive and Gram-negative bacteria. The minimum inhibitory concentration (MIC) values for THQA against common pathogens are noteworthy:
Pathogen | MIC (µg/mL) |
---|---|
Staphylococcus aureus | 50 |
Escherichia coli | 75 |
Pseudomonas aeruginosa | 100 |
These results highlight the potential of THQA as an antimicrobial agent, particularly in combating infections caused by resistant strains .
Anticancer Activity
THQA has also been investigated for its anticancer properties. Studies have shown that it can inhibit the proliferation of various cancer cell lines, including:
Cell Line | IC50 (µM) |
---|---|
HeLa (cervical cancer) | 12.5 |
A549 (lung cancer) | 15.0 |
MCF-7 (breast cancer) | 18.5 |
The mechanism underlying its anticancer activity may involve the induction of apoptosis and disruption of cell cycle progression .
Case Studies and Research Findings
A notable case study examined the effects of THQA on human cervical cancer cells (HeLa). The study found that treatment with THQA resulted in a significant reduction in cell viability, coupled with increased markers of apoptosis such as caspase activation and PARP cleavage. Additionally, THQA was shown to downregulate anti-apoptotic proteins while upregulating pro-apoptotic factors .
Another research effort focused on the compound's neuroprotective effects. In models of neurodegeneration, THQA demonstrated the ability to protect neuronal cells from oxidative stress-induced apoptosis, suggesting its potential application in treating neurodegenerative diseases .
Comparative Analysis with Similar Compounds
THQA shares structural similarities with other quinoxaline derivatives but exhibits unique biological properties due to its specific functional groups. A comparison with similar compounds reveals distinct differences in biological activity:
Compound | Activity Type | Notable Effects |
---|---|---|
2-Quinoxalineacetic acid | Antimicrobial | Moderate activity against E. coli |
1,2,3,4-Tetrahydro-3-isoquinolinecarboxylic acid | Anticancer | Limited efficacy compared to THQA |
2-Oxo-1,2,3,4-tetrahydroquinolinecarboxylic acid | Neuroprotective | Less effective than THQA |
This table illustrates how THQA stands out among its peers in terms of potency and range of biological activities .
Propriétés
IUPAC Name |
2-(3-oxo-2,4-dihydro-1H-quinoxalin-2-yl)acetic acid | |
---|---|---|
Source | PubChem | |
URL | https://pubchem.ncbi.nlm.nih.gov | |
Description | Data deposited in or computed by PubChem | |
InChI |
InChI=1S/C10H10N2O3/c13-9(14)5-8-10(15)12-7-4-2-1-3-6(7)11-8/h1-4,8,11H,5H2,(H,12,15)(H,13,14) | |
Source | PubChem | |
URL | https://pubchem.ncbi.nlm.nih.gov | |
Description | Data deposited in or computed by PubChem | |
InChI Key |
UCNFCRXGPQHQBE-UHFFFAOYSA-N | |
Source | PubChem | |
URL | https://pubchem.ncbi.nlm.nih.gov | |
Description | Data deposited in or computed by PubChem | |
Canonical SMILES |
C1=CC=C2C(=C1)NC(C(=O)N2)CC(=O)O | |
Source | PubChem | |
URL | https://pubchem.ncbi.nlm.nih.gov | |
Description | Data deposited in or computed by PubChem | |
Molecular Formula |
C10H10N2O3 | |
Source | PubChem | |
URL | https://pubchem.ncbi.nlm.nih.gov | |
Description | Data deposited in or computed by PubChem | |
DSSTOX Substance ID |
DTXSID00382712 | |
Record name | (3-Oxo-1,2,3,4-tetrahydroquinoxalin-2-yl)acetic acid | |
Source | EPA DSSTox | |
URL | https://comptox.epa.gov/dashboard/DTXSID00382712 | |
Description | DSSTox provides a high quality public chemistry resource for supporting improved predictive toxicology. | |
Molecular Weight |
206.20 g/mol | |
Source | PubChem | |
URL | https://pubchem.ncbi.nlm.nih.gov | |
Description | Data deposited in or computed by PubChem | |
CAS No. |
136584-14-0 | |
Record name | (3-Oxo-1,2,3,4-tetrahydroquinoxalin-2-yl)acetic acid | |
Source | EPA DSSTox | |
URL | https://comptox.epa.gov/dashboard/DTXSID00382712 | |
Description | DSSTox provides a high quality public chemistry resource for supporting improved predictive toxicology. | |
Synthesis routes and methods
Procedure details
Avertissement et informations sur les produits de recherche in vitro
Veuillez noter que tous les articles et informations sur les produits présentés sur BenchChem sont destinés uniquement à des fins informatives. Les produits disponibles à l'achat sur BenchChem sont spécifiquement conçus pour des études in vitro, qui sont réalisées en dehors des organismes vivants. Les études in vitro, dérivées du terme latin "in verre", impliquent des expériences réalisées dans des environnements de laboratoire contrôlés à l'aide de cellules ou de tissus. Il est important de noter que ces produits ne sont pas classés comme médicaments et n'ont pas reçu l'approbation de la FDA pour la prévention, le traitement ou la guérison de toute condition médicale, affection ou maladie. Nous devons souligner que toute forme d'introduction corporelle de ces produits chez les humains ou les animaux est strictement interdite par la loi. Il est essentiel de respecter ces directives pour assurer la conformité aux normes légales et éthiques en matière de recherche et d'expérimentation.