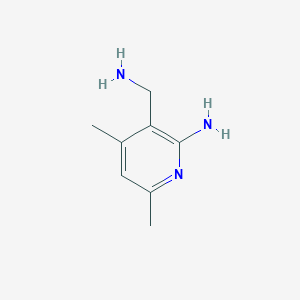
3-(Aminomethyl)-4,6-dimethylpyridin-2-amine
Vue d'ensemble
Description
3-(Aminomethyl)-4,6-dimethylpyridin-2-amine is an organic compound that belongs to the class of aminomethyl derivatives of pyridine. This compound features a pyridine ring substituted with aminomethyl and dimethyl groups, making it a versatile molecule in various chemical reactions and applications.
Méthodes De Préparation
Synthetic Routes and Reaction Conditions
The synthesis of 3-(Aminomethyl)-4,6-dimethylpyridin-2-amine typically involves multi-step organic reactions. One common method includes the alkylation of 4,6-dimethylpyridin-2-amine with formaldehyde and subsequent reduction. The reaction conditions often require a base such as sodium hydroxide and a reducing agent like sodium borohydride.
Industrial Production Methods
In an industrial setting, the production of this compound can be scaled up using continuous flow reactors to ensure consistent quality and yield. The process involves the same basic steps but optimized for large-scale production, including precise control of temperature, pressure, and reactant concentrations.
Analyse Des Réactions Chimiques
Types of Reactions
3-(Aminomethyl)-4,6-dimethylpyridin-2-amine undergoes various chemical reactions, including:
Oxidation: The compound can be oxidized using agents like potassium permanganate.
Reduction: Reduction reactions can be performed using hydrogen gas in the presence of a palladium catalyst.
Substitution: Nucleophilic substitution reactions are common, where the aminomethyl group can be replaced by other nucleophiles.
Common Reagents and Conditions
Oxidation: Potassium permanganate in an acidic medium.
Reduction: Hydrogen gas with palladium on carbon as a catalyst.
Substitution: Alkyl halides or acyl chlorides in the presence of a base.
Major Products
Oxidation: Produces corresponding pyridine N-oxides.
Reduction: Leads to the formation of reduced pyridine derivatives.
Substitution: Results in various substituted pyridine compounds.
Applications De Recherche Scientifique
Medicinal Chemistry Applications
1.1 Dual Inhibitor Development
Recent studies have identified 3-(Aminomethyl)-4,6-dimethylpyridin-2-amine as a promising candidate for developing dual inhibitors targeting EZH2 and HSP90. One notable study demonstrated that derivatives of this compound exhibited significant inhibitory effects on cell growth in TMZ-resistant glioblastoma cell lines. The compound was incorporated into various molecular frameworks to enhance its potency and selectivity.
Case Study: EZH2-HSP90 Inhibitor
- Objective: To evaluate the efficacy of a dual inhibitor in glioblastoma treatment.
- Methodology: The compound was synthesized and tested against multiple glioblastoma cell lines.
- Results:
Table 1: Cell Growth Inhibition Data
Compound | IC50 (μM) |
---|---|
Dual Inhibitor 1 | 1.015 ± 0.69 |
Dual Inhibitor 2 | 10.83 ± 1 |
Tazemetostat (control) | 79.81 ± 4.91 |
This compound has been linked to various biological activities beyond anticancer properties:
- Antifungal Activity: Exhibited effectiveness against certain fungal strains.
- Antibacterial Properties: Demonstrated inhibition of bacterial growth in preliminary studies.
- Antiviral Potential: Investigated for activity against viral infections.
These properties make it a versatile candidate for further pharmacological exploration.
Organic Synthesis Applications
The compound serves as a valuable intermediate in organic synthesis, particularly in creating complex molecular architectures:
3.1 Synthesis of Novel Compounds
In synthetic chemistry, this compound has been utilized as a building block for synthesizing various derivatives with enhanced biological activities:
Example Synthesis Pathway:
- Starting Material: 3-(Aminomethyl)-4,6-dimethylpyridin-2(1H)-one
- Reagents: Triethylamine, EDC-HCl
- Conditions: Reactions conducted in dimethyl sulfoxide at controlled temperatures.
This pathway has led to the development of several novel compounds with improved pharmacological profiles .
Structural Insights and Computational Studies
Computational studies have provided insights into the molecular interactions and potential binding sites of this compound with biological targets:
4.1 Molecular Docking Studies
Molecular docking simulations have been performed to predict how this compound interacts with specific enzymes and receptors involved in cancer pathways:
- Findings: The compound showed favorable binding affinities with EZH2 and HSP90, supporting its role as an inhibitor.
Table 2: Docking Results Summary
Target Enzyme | Binding Affinity (kcal/mol) |
---|---|
EZH2 | -9.5 |
HSP90 | -8.7 |
Mécanisme D'action
The mechanism of action of 3-(Aminomethyl)-4,6-dimethylpyridin-2-amine involves its interaction with specific molecular targets, such as enzymes or receptors. The aminomethyl group can form hydrogen bonds with active sites, while the pyridine ring can participate in π-π interactions, influencing the compound’s biological activity.
Comparaison Avec Des Composés Similaires
Similar Compounds
- 3-(Aminomethyl)-PROXYL
- N-[3-(aminomethyl)benzyl]acetamidine
- Muscimol
Uniqueness
3-(Aminomethyl)-4,6-dimethylpyridin-2-amine is unique due to its specific substitution pattern on the pyridine ring, which imparts distinct chemical and biological properties. Compared to similar compounds, it offers a unique combination of reactivity and stability, making it valuable in various applications.
Activité Biologique
3-(Aminomethyl)-4,6-dimethylpyridin-2-amine, also known as 3-AP, is a pyridine derivative that has garnered attention for its potential biological activities. This compound is characterized by the presence of an amino group and two methyl groups on the pyridine ring, which may influence its pharmacological properties. This article will explore the biological activity of 3-AP, including its mechanisms of action, therapeutic applications, and relevant research findings.
Chemical Structure and Properties
The chemical structure of this compound can be represented as follows:
- Molecular Formula: C8H12N2
- Molecular Weight: 152.19 g/mol
- CAS Number: 7063844
The compound features a pyridine ring substituted with an aminomethyl group at the 3-position and methyl groups at the 4 and 6 positions. This unique structure may contribute to its biological activity.
Research indicates that 3-AP exhibits various biological activities through multiple mechanisms:
- Enzyme Inhibition : It has been shown to inhibit specific enzymes involved in metabolic pathways, potentially affecting cellular processes such as proliferation and apoptosis.
- Receptor Interaction : The compound may interact with neurotransmitter receptors, influencing signaling pathways related to mood regulation and cognitive function.
- Antioxidant Properties : Preliminary studies suggest that 3-AP may possess antioxidant activity, helping to mitigate oxidative stress in cells.
Therapeutic Applications
The potential therapeutic applications of this compound include:
- Anticancer Activity : Some studies have explored its role in inhibiting cancer cell growth. For instance, research has indicated that derivatives of pyridine compounds can exhibit cytotoxic effects against various cancer cell lines.
- Neuroprotective Effects : Given its interaction with neurotransmitter systems, there is interest in studying its effects on neurodegenerative diseases.
Case Studies and Research Findings
Table 1: Biological Activities of this compound
Table 2: Comparative Analysis of Pyridine Derivatives
Propriétés
IUPAC Name |
3-(aminomethyl)-4,6-dimethylpyridin-2-amine | |
---|---|---|
Source | PubChem | |
URL | https://pubchem.ncbi.nlm.nih.gov | |
Description | Data deposited in or computed by PubChem | |
InChI |
InChI=1S/C8H13N3/c1-5-3-6(2)11-8(10)7(5)4-9/h3H,4,9H2,1-2H3,(H2,10,11) | |
Source | PubChem | |
URL | https://pubchem.ncbi.nlm.nih.gov | |
Description | Data deposited in or computed by PubChem | |
InChI Key |
YIBATIFWEVKVHL-UHFFFAOYSA-N | |
Source | PubChem | |
URL | https://pubchem.ncbi.nlm.nih.gov | |
Description | Data deposited in or computed by PubChem | |
Canonical SMILES |
CC1=CC(=NC(=C1CN)N)C | |
Source | PubChem | |
URL | https://pubchem.ncbi.nlm.nih.gov | |
Description | Data deposited in or computed by PubChem | |
Molecular Formula |
C8H13N3 | |
Source | PubChem | |
URL | https://pubchem.ncbi.nlm.nih.gov | |
Description | Data deposited in or computed by PubChem | |
DSSTOX Substance ID |
DTXSID50542864 | |
Record name | 3-(Aminomethyl)-4,6-dimethylpyridin-2-amine | |
Source | EPA DSSTox | |
URL | https://comptox.epa.gov/dashboard/DTXSID50542864 | |
Description | DSSTox provides a high quality public chemistry resource for supporting improved predictive toxicology. | |
Molecular Weight |
151.21 g/mol | |
Source | PubChem | |
URL | https://pubchem.ncbi.nlm.nih.gov | |
Description | Data deposited in or computed by PubChem | |
CAS No. |
96551-20-1 | |
Record name | 3-(Aminomethyl)-4,6-dimethylpyridin-2-amine | |
Source | EPA DSSTox | |
URL | https://comptox.epa.gov/dashboard/DTXSID50542864 | |
Description | DSSTox provides a high quality public chemistry resource for supporting improved predictive toxicology. | |
Avertissement et informations sur les produits de recherche in vitro
Veuillez noter que tous les articles et informations sur les produits présentés sur BenchChem sont destinés uniquement à des fins informatives. Les produits disponibles à l'achat sur BenchChem sont spécifiquement conçus pour des études in vitro, qui sont réalisées en dehors des organismes vivants. Les études in vitro, dérivées du terme latin "in verre", impliquent des expériences réalisées dans des environnements de laboratoire contrôlés à l'aide de cellules ou de tissus. Il est important de noter que ces produits ne sont pas classés comme médicaments et n'ont pas reçu l'approbation de la FDA pour la prévention, le traitement ou la guérison de toute condition médicale, affection ou maladie. Nous devons souligner que toute forme d'introduction corporelle de ces produits chez les humains ou les animaux est strictement interdite par la loi. Il est essentiel de respecter ces directives pour assurer la conformité aux normes légales et éthiques en matière de recherche et d'expérimentation.