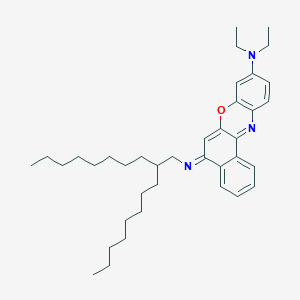
Chromoionophore III
Vue d'ensemble
Description
Chromoionophore III, also known as 9-(Diethylamino)-5-[(2-octyldecyl)imino]benzo[a]phenoxazine, is a chemical compound with the empirical formula C38H55N3O and a molecular weight of 569.86 g/mol . It is a member of the chromoionophore family, which are compounds known for their ability to change color in response to ion binding. This property makes this compound particularly useful in various sensing applications.
Méthodes De Préparation
Synthetic Routes and Reaction Conditions
The synthesis of Chromoionophore III typically involves the reaction of 9-diethylaminobenzo[a]phenoxazine with an appropriate alkylating agent to introduce the 2-octyldecyl group. The reaction conditions often include the use of a solvent such as dichloromethane and a base like potassium carbonate to facilitate the alkylation process .
Industrial Production Methods
Industrial production methods for this compound are not extensively documented in the public domain. it is likely that the process involves similar synthetic routes as those used in laboratory settings, scaled up to accommodate larger production volumes. The use of continuous flow reactors and automated synthesis platforms may be employed to enhance efficiency and yield.
Analyse Des Réactions Chimiques
Types of Reactions
Chromoionophore III primarily undergoes reactions that involve its ionophore properties. These include:
Complexation Reactions: This compound can form complexes with various ions, leading to changes in its optical properties.
Substitution Reactions: The compound can undergo substitution reactions where the diethylamino group or the octyldecyl group is replaced by other functional groups.
Common Reagents and Conditions
Major Products
The major products formed from reactions involving this compound depend on the specific reaction conditions and reagents used. For example, alkylation reactions will yield derivatives with different alkyl groups, while complexation reactions will result in ion-bound complexes .
Applications De Recherche Scientifique
Chromoionophore III has a wide range of applications in scientific research, particularly in the fields of chemistry, biology, and medicine:
Mécanisme D'action
Chromoionophore III exerts its effects through its ability to bind selectively to specific ions. The binding of an ion to this compound induces a conformational change in the molecule, leading to a shift in its optical properties, such as color or fluorescence . This change can be detected and measured, allowing for the quantification of ion concentrations in various environments.
Comparaison Avec Des Composés Similaires
Chromoionophore III is unique among chromoionophores due to its specific structure and ion-binding properties. Similar compounds include:
Chromoionophore I: Known for its lower pKa value and ability to detect ions at physiological concentrations.
Chromoionophore II: Another member of the chromoionophore family with different ion selectivity and optical properties.
Compared to these compounds, this compound offers distinct advantages in terms of its binding affinity and optical response, making it particularly suitable for applications requiring high sensitivity and specificity .
Activité Biologique
Chromoionophore III (CHIII) is a specialized compound used primarily in the development of optical nanosensors for monitoring various ions and biomolecules in biological systems. This article reviews the biological activity of CHIII, focusing on its mechanisms, applications, and research findings.
Overview of this compound
This compound is a fluorescent ion-selective compound that has been utilized in various sensor technologies. Its ability to selectively bind ions and change fluorescence properties makes it particularly valuable in biological imaging and sensing applications. The compound is often integrated into nanosensors that can detect changes in ion concentrations within cells, providing insights into cellular processes.
The biological activity of CHIII is largely attributed to its ion-selective properties. When CHIII binds to specific ions, it undergoes conformational changes that alter its fluorescence characteristics. This property is exploited in ratiometric sensing, where the ratio of fluorescence intensities from CHIII and another fluorophore (e.g., octadecyl rhodamine) is used to quantify ion concentrations.
Key Mechanisms:
- Ion Binding: CHIII exhibits selective binding for certain cations (e.g., Na, K) and anions, which leads to changes in its optical properties.
- Fluorescence Resonance Energy Transfer (FRET): The interaction between CHIII and other fluorophores can result in energy transfer processes that enhance detection sensitivity.
- pH Sensitivity: The pKa of CHIII influences its performance in varying pH environments, making it suitable for applications in different cellular contexts.
Ion Selectivity and Sensitivity
Recent studies have highlighted the effectiveness of CHIII in detecting ion concentrations relevant to physiological conditions. For instance, research demonstrated that CHIII maintains sensitivity over a range of sodium concentrations (10 mM to 100 mM) with minimal degradation over time .
Ion | Concentration Range | Sensitivity | Fluorescence Change |
---|---|---|---|
Sodium (Na) | 10 mM - 100 mM | High | Decreased by 40% |
Potassium (K) | Variable | Moderate | Significant change noted |
Case Studies
-
Cell Imaging Applications:
In a study involving HeLa cells, CHIII was incorporated into nanoparticle-based sensors which were successfully endocytosed by the cells. The sensors demonstrated ratiometric pH responses, indicating their potential for real-time intracellular monitoring . -
Histamine Detection:
Another study focused on using CHIII for histamine detection in vitro. The nanosensors showed effective calibration against varying histamine concentrations, although initial tests indicated a need for reformulation to enhance sensitivity at lower concentrations . -
Long-term Stability:
An investigation into the long-term stability of CHIII revealed that it retained its sensitivity over an eight-day period when exposed to physiological conditions. This stability is crucial for applications requiring prolonged monitoring .
Limitations and Challenges
Despite its promising applications, the use of CHIII is not without challenges:
- Endosomal Escape: The ability of nanosensors containing CHIII to escape endosomal compartments remains limited, potentially affecting measurement accuracy .
- Ion Interference: Variability in ion concentrations can lead to interference, complicating the interpretation of fluorescence signals .
- Calibration Needs: Continuous calibration may be required to maintain accuracy across different biological environments .
Propriétés
IUPAC Name |
N,N-diethyl-5-(2-octyldecylimino)benzo[a]phenoxazin-9-amine | |
---|---|---|
Source | PubChem | |
URL | https://pubchem.ncbi.nlm.nih.gov | |
Description | Data deposited in or computed by PubChem | |
InChI |
InChI=1S/C38H55N3O/c1-5-9-11-13-15-17-21-30(22-18-16-14-12-10-6-2)29-39-35-28-37-38(33-24-20-19-23-32(33)35)40-34-26-25-31(27-36(34)42-37)41(7-3)8-4/h19-20,23-28,30H,5-18,21-22,29H2,1-4H3 | |
Source | PubChem | |
URL | https://pubchem.ncbi.nlm.nih.gov | |
Description | Data deposited in or computed by PubChem | |
InChI Key |
ICVAQSQRYBGWQH-UHFFFAOYSA-N | |
Source | PubChem | |
URL | https://pubchem.ncbi.nlm.nih.gov | |
Description | Data deposited in or computed by PubChem | |
Canonical SMILES |
CCCCCCCCC(CCCCCCCC)CN=C1C=C2C(=NC3=C(O2)C=C(C=C3)N(CC)CC)C4=CC=CC=C41 | |
Source | PubChem | |
URL | https://pubchem.ncbi.nlm.nih.gov | |
Description | Data deposited in or computed by PubChem | |
Molecular Formula |
C38H55N3O | |
Source | PubChem | |
URL | https://pubchem.ncbi.nlm.nih.gov | |
Description | Data deposited in or computed by PubChem | |
DSSTOX Substance ID |
DTXSID10402894 | |
Record name | Chromoionophore III | |
Source | EPA DSSTox | |
URL | https://comptox.epa.gov/dashboard/DTXSID10402894 | |
Description | DSSTox provides a high quality public chemistry resource for supporting improved predictive toxicology. | |
Molecular Weight |
569.9 g/mol | |
Source | PubChem | |
URL | https://pubchem.ncbi.nlm.nih.gov | |
Description | Data deposited in or computed by PubChem | |
CAS No. |
149683-18-1 | |
Record name | Chromoionophore III | |
Source | EPA DSSTox | |
URL | https://comptox.epa.gov/dashboard/DTXSID10402894 | |
Description | DSSTox provides a high quality public chemistry resource for supporting improved predictive toxicology. | |
Retrosynthesis Analysis
AI-Powered Synthesis Planning: Our tool employs the Template_relevance Pistachio, Template_relevance Bkms_metabolic, Template_relevance Pistachio_ringbreaker, Template_relevance Reaxys, Template_relevance Reaxys_biocatalysis model, leveraging a vast database of chemical reactions to predict feasible synthetic routes.
One-Step Synthesis Focus: Specifically designed for one-step synthesis, it provides concise and direct routes for your target compounds, streamlining the synthesis process.
Accurate Predictions: Utilizing the extensive PISTACHIO, BKMS_METABOLIC, PISTACHIO_RINGBREAKER, REAXYS, REAXYS_BIOCATALYSIS database, our tool offers high-accuracy predictions, reflecting the latest in chemical research and data.
Strategy Settings
Precursor scoring | Relevance Heuristic |
---|---|
Min. plausibility | 0.01 |
Model | Template_relevance |
Template Set | Pistachio/Bkms_metabolic/Pistachio_ringbreaker/Reaxys/Reaxys_biocatalysis |
Top-N result to add to graph | 6 |
Feasible Synthetic Routes
Avertissement et informations sur les produits de recherche in vitro
Veuillez noter que tous les articles et informations sur les produits présentés sur BenchChem sont destinés uniquement à des fins informatives. Les produits disponibles à l'achat sur BenchChem sont spécifiquement conçus pour des études in vitro, qui sont réalisées en dehors des organismes vivants. Les études in vitro, dérivées du terme latin "in verre", impliquent des expériences réalisées dans des environnements de laboratoire contrôlés à l'aide de cellules ou de tissus. Il est important de noter que ces produits ne sont pas classés comme médicaments et n'ont pas reçu l'approbation de la FDA pour la prévention, le traitement ou la guérison de toute condition médicale, affection ou maladie. Nous devons souligner que toute forme d'introduction corporelle de ces produits chez les humains ou les animaux est strictement interdite par la loi. Il est essentiel de respecter ces directives pour assurer la conformité aux normes légales et éthiques en matière de recherche et d'expérimentation.