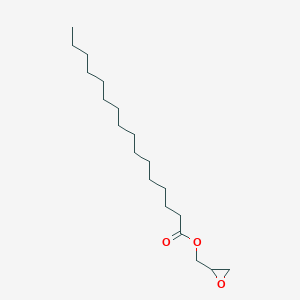
Glycidyl Palmitate
Vue d'ensemble
Description
Glycidyl palmitate (C₁₉H₃₆O₃, CAS 7501-44-2) is a glycidyl ester derived from palmitic acid (C16:0). It has a molecular weight of 312.49 g/mol, a logP value of 5.41 (indicating high lipophilicity), and a melting point of 39–41°C . It is commonly detected in refined vegetable oils, baked goods, and fried foods, where it forms during high-temperature processing via the degradation of diacylglycerols or triglycerides .
Méthodes De Préparation
Voies de synthèse et conditions de réaction : Le palmitate de glycidyle peut être synthétisé par l'estérification du glycidol avec l'acide palmitique. Cette réaction nécessite généralement un catalyseur, tel que l'acide sulfurique, et est effectuée sous des conditions de température contrôlées pour assurer la formation de la liaison ester sans réactions secondaires indésirables .
Méthodes de production industrielle : Dans les environnements industriels, le palmitate de glycidyle est souvent produit lors du raffinage des huiles végétales. Les températures élevées utilisées lors de l'étape de désodorisation du raffinage des huiles peuvent conduire à la formation d'esters de glycidyle, y compris le palmitate de glycidyle . Le processus implique la réaction de mono- et diacylglycérols avec du glycidol dans ces conditions.
Analyse Des Réactions Chimiques
Types de réactions : Le palmitate de glycidyle subit plusieurs types de réactions chimiques, notamment :
Hydrolyse : La liaison ester peut être hydrolysée en présence d'eau et d'un catalyseur acide ou basique, conduisant à la formation de glycidol et d'acide palmitique.
Ouverture du cycle époxyde : Le groupe époxyde dans le palmitate de glycidyle est très réactif et peut subir des réactions d'ouverture de cycle avec des nucléophiles tels que l'eau, les alcools et les amines.
Réactifs et conditions courants :
Hydrolyse : Généralement réalisée avec de l'acide chlorhydrique ou de l'hydroxyde de sodium comme catalyseur.
Ouverture du cycle époxyde : Les nucléophiles courants comprennent l'eau, le méthanol et l'ammoniac, souvent dans des conditions de température douce.
Principaux produits formés :
Hydrolyse : Glycidol et acide palmitique.
Ouverture du cycle époxyde : Selon le nucléophile, les produits peuvent inclure des dérivés du glycérol et divers alcools ou amines.
4. Applications de la recherche scientifique
Le palmitate de glycidyle a plusieurs applications dans la recherche scientifique :
Chimie : Utilisé comme composé modèle pour étudier le comportement des esters de glycidyle et leurs réactions.
Biologie : Étudié pour ses effets potentiels sur les systèmes biologiques, en particulier ses interactions avec les enzymes et les composants cellulaires.
5. Mécanisme d'action
Le principal mécanisme d'action du palmitate de glycidyle implique la réactivité de son groupe époxyde. Ce groupe peut former des liaisons covalentes avec des sites nucléophiles dans les molécules biologiques, telles que les protéines et l'ADN. Cette réactivité peut conduire à la modification de ces molécules, affectant potentiellement leur fonction et conduisant à divers effets biologiques . Les cibles moléculaires exactes et les voies impliquées dépendent du contexte spécifique et des conditions d'exposition.
Composés similaires :
Stéarate de glycidyle : Un autre ester de glycidyle formé à partir du glycidol et de l'acide stéarique. Il partage une réactivité et des applications similaires, mais diffère par le composant acide gras.
Unicité du palmitate de glycidyle : Le palmitate de glycidyle est unique en raison de son composant acide gras spécifique, l'acide palmitique, qui est un acide gras saturé. Cela lui confère des propriétés physiques et chimiques distinctes par rapport aux esters de glycidyle avec des acides gras insaturés. Sa formation lors du raffinage de l'huile de palme et d'autres huiles végétales le rend également particulièrement pertinent dans le contexte de la sécurité alimentaire et des procédés industriels .
Applications De Recherche Scientifique
Scientific Research Applications
Glycidyl palmitate has diverse applications across several scientific fields, including chemistry, biology, medicine, and industry.
Chemistry
- Model Compound : this compound serves as a model compound for studying glycidyl esters' behavior and their chemical reactions. Researchers utilize it to understand the reactivity patterns of similar compounds and to develop new synthetic methodologies.
Biology
- Lipid Modification Studies : The compound is investigated for its role in lipid modifications, particularly S-palmitoylation, which is critical for protein membrane association and cellular signaling processes. Studies have shown that this compound can influence cell function by altering the membrane affinity of proteins, thereby affecting signaling pathways and gene expression.
Medicine
- Drug Delivery Systems : this compound is explored for potential applications in drug delivery systems due to its ability to form stable complexes with various drugs. This property may enhance the bioavailability and therapeutic efficacy of certain medications.
Industry
- Surfactants and Emulsifiers : In industrial applications, this compound is used in producing surfactants and emulsifiers essential for formulating cosmetics and personal care products. Its properties make it suitable for stabilizing emulsions and enhancing product textures.
Direct Analysis
A study developed an LC-MS method for the direct determination of glycidyl esters in vegetable oils, including this compound. The method demonstrated high reproducibility and sensitivity, allowing for accurate quantification of glycidyl esters at low concentrations .
Elimination Techniques
Research has also focused on eliminating this compound from edible oils using activated bleaching earth (ABE). This method showed that ABE could effectively remove glycidyl esters through a transformation process involving ring-opening reactions followed by interesterification, achieving a recovery rate of over 99% .
Case Study 1: Toxicological Assessment
In a toxicological study, the dietary exposure to glycidyl esters like this compound raised health concerns due to their potential conversion into toxic compounds in the gastrointestinal tract. The International Agency for Research on Cancer (IARC) has classified glycidol (a hydrolysis product) as a possible human carcinogen, highlighting the importance of monitoring these compounds in food products .
Case Study 2: Industrial Application
In industrial settings, this compound's formation during the deodorization of vegetable oils has been studied extensively. Researchers have examined how refining conditions impact the levels of glycidyl esters in oils and have developed methods to minimize their formation during processing to ensure food safety .
Mécanisme D'action
The primary mechanism of action of glycidyl palmitate involves the reactivity of its epoxide group. This group can form covalent bonds with nucleophilic sites in biological molecules, such as proteins and DNA. This reactivity can lead to the modification of these molecules, potentially affecting their function and leading to various biological effects . The exact molecular targets and pathways involved depend on the specific context and conditions of exposure.
Comparaison Avec Des Composés Similaires
Structural and Physicochemical Comparison with Similar Glycidyl Esters
Glycidyl palmitate belongs to a homologous series of glycidyl esters differing in fatty acid chain length and saturation. Key structural and analytical properties are compared below:
Table 1: Structural and Analytical Properties of Glycidyl Esters
Compound | Formula | Molecular Weight (g/mol) | [M + H]⁺ (m/z) | LogP* | Method LOQ (ng/g) |
---|---|---|---|---|---|
Glycidyl laurate | C₁₅H₂₈O₃ | 256.35 | 257.2 | ~4.5 | 200 |
Glycidyl myristate | C₁₇H₃₂O₃ | 284.43 | 285.2 | ~5.0 | 200 |
This compound | C₁₉H₃₆O₃ | 312.49 | 313.3 | 5.41 | 200 |
Glycidyl stearate | C₂₁H₄₀O₃ | 340.54 | 341.3 | ~6.0 | 600 |
Glycidyl oleate | C₂₁H₃₈O₃ | 338.53 | 339.3 | ~5.8 | 300 |
Glycidyl linoleate | C₂₁H₃₆O₃ | 336.51 | 337.3 | ~5.5 | 200 |
*Estimated logP values based on chain length and saturation .
Key Observations :
- Molecular weight and hydrophobicity increase with fatty acid chain length (e.g., laurate [C12] to stearate [C18:0]).
- Detection limits vary: Stearate and oleate have higher LOQs (600 and 300 ng/g, respectively) due to lower volatility and ionization efficiency in LC-MS .
- Saturation effects: Unsaturated esters (e.g., oleate [C18:1], linoleate [C18:2]) exhibit lower molecular weights and slightly reduced logP values compared to saturated analogs.
Occurrence in Food Matrices
Glycidyl esters form during thermal processing of oils and fats. Their abundance depends on the fatty acid profile of the source oil and processing conditions:
Table 2: Relative Abundance in Heated Foods*
Food Matrix | Most Abundant Ester (%) | This compound (%) | Glycidyl Stearate (%) | Glycidyl Oleate (%) |
---|---|---|---|---|
Beef (600°C, 5 min) | Oleate (51.6) | 18.9 | 12.3 | 51.6 |
Pork patties (500°C) | Oleate (55.4) | 15.2 | 10.1 | 55.4 |
Cupcakes (210°C) | Oleate (76.6) | 76.6 |
*Data from ; LOD = Limit of Detection.
Key Findings :
- Glycidyl oleate dominates in most matrices due to the prevalence of oleic acid in edible oils .
- This compound is prominent in palm oil-derived products but often undetectable in baked goods (e.g., cupcakes) due to lower palmitic acid content in non-tropical oils .
- Matrix effects : Detection depends on analytical methods. For example, LC-MS with deuterated internal standards improves accuracy for palmitate and stearate .
Hydrolysis and Toxicity
Glycidyl esters are hydrolyzed by lipases to release glycidol. Studies in rats show this compound is fully hydrolyzed, yielding glycidol and palmitic acid . However, species-specific lipase activity affects hydrolysis rates; crab-eating macaques show slower conversion than rats due to lower gastric lipase activity .
Antimicrobial Activity
Both this compound and oleate exhibit antimicrobial properties, likely due to their surfactant-like disruption of microbial membranes . No comparative efficacy data are available for other esters.
Mitigation Strategies
Activated carbon (AC) effectively removes glycidyl esters from oils. Acid-washed oil palm AC achieves >95% removal of this compound via adsorption, following a Langmuir isotherm model . The efficiency for other esters remains unstudied but is expected to correlate with hydrophobicity (higher logP = better adsorption).
Activité Biologique
Glycidyl palmitate (GP) is a synthetic fatty acid ester that has garnered attention due to its biological activity, particularly in the context of food safety, antioxidant properties, and potential health implications. This article synthesizes recent research findings on GP, focusing on its biological activity, mechanisms of action, and implications for human health.
This compound is formed by the reaction of palmitic acid with epichlorohydrin. It is primarily used in the production of polylactic acid and other biodegradable polymers, making it relevant in various industrial applications . Its structure includes reactive hydroxyl groups that can participate in various biochemical reactions.
Antioxidant and Anti-Inflammatory Properties
Recent studies have highlighted the antioxidant and anti-inflammatory properties of this compound. In a study examining the lipophilic fraction of Liriope platyphylla seeds, GP was identified as one of the top active compounds exhibiting significant antioxidant activity. This activity was assessed through various chemical assays measuring scavenging effects on free radicals such as DPPH and ABTS .
The mechanism behind these properties involves the regulation of several key signaling pathways and genes associated with inflammation and oxidative stress. Specifically, GP influences targets such as NFKB1, PTGS1, PTGS2, and TLR4, which are crucial in mediating inflammatory responses .
Hydrolysis and Transformation
This compound undergoes hydrolysis by lipase enzymes, resulting in the formation of glycidol and palmitic acid. This transformation is significant because glycidol has been associated with genotoxic effects. The hydrolysis process can be monitored using gas-liquid chromatography to assess the efficiency of GP removal from oils during processing .
Study on Food Processing Contaminants
A comprehensive assessment of glycidyl esters, including GP, was conducted in relation to their formation during thermal processing of foods. The study found that glycidol can react with DNA bases, leading to the formation of glycidyl-DNA adducts, which serve as biomarkers for exposure to glycidol . Such findings raise concerns about the potential carcinogenic effects of GP when present in heated food products.
Elimination Techniques
In another study focused on food safety, activated bleaching earth (ABE) was used to eliminate glycidyl esters from diacylglycerol oils. The results indicated that GP could be effectively transformed into less harmful compounds through treatment with ABE, showcasing a method for reducing potential health risks associated with its consumption .
Research Findings Summary Table
Q & A
Basic Research Questions
Q. What are the key physicochemical properties of Glycidyl Palmitate relevant to its analysis in lipid matrices?
this compound (C₁₉H₃₆O₃; molecular weight 312.49 g/mol) exhibits a high LogP value (5.41), indicating strong hydrophobicity, which influences its partitioning into lipid matrices. Its melting point (39–41°C) and density (0.8889 g/cm³ at 60°C) are critical for solubility considerations in analytical workflows. Sparing solubility in chloroform and slight solubility in ethyl acetate necessitate solvent optimization for extraction and chromatography .
Q. How is this compound detected and quantified in complex food matrices?
Liquid chromatography–mass spectrometry (LC-MS) and gas chromatography–mass spectrometry (GC-MS) are primary methods. Deuterated internal standards (e.g., this compound-d5) are used to correct for matrix effects and ionization variability. Calibration curves are constructed using certified standards (purity ≥98%), with detection limits as low as ng/g levels in baked goods and oils .
Q. What analytical challenges arise when distinguishing this compound from 3-MCPD esters?
Structural similarities between this compound and 3-MCPD esters (e.g., 1,2-dipalmitoyl-3-chloropropanediol) require selective derivatization or chromatographic separation. Phenylboronic acid (PBA) derivatization followed by GC-MS in selected ion monitoring (SIM) or multiple reaction monitoring (MRM) modes enhances specificity. Cross-validation with LC-MS/MS is recommended to resolve co-elution issues .
Advanced Research Questions
Q. How do thermal processing conditions influence this compound formation kinetics in edible oils?
Formation is temperature-dependent and varies by matrix. In rapeseed oil heated at 170–250°C, this compound levels remain below detection limits, while glycidyl oleate dominates (62–72% of total GEs). In contrast, meat patties heated at 500–600°C in a muffle furnace show detectable this compound, with increasing yields at higher temperatures and prolonged durations. Oleic acid-rich matrices favor glycidyl oleate formation due to fatty acid specificity .
Q. What adsorption mechanisms effectively remove this compound from oil matrices?
Acid-washed oil palm wood-based activated carbon (OPAC) achieves >95% removal via physisorption, fitting the Langmuir model (max capacity: 36.23 mg/g). Process efficiency depends on contact time (equilibrium at ~120 min), adsorbent dose, and temperature (endothermic process). Competitive adsorption with other glycidyl esters (e.g., stearate, linoleate) may reduce efficacy, necessitating matrix-specific optimization .
Q. How do deuterated analogs (e.g., this compound-d5) enhance quantification accuracy in mass spectrometry?
Deuterated standards compensate for ion suppression/enhancement and extraction losses. For example, this compound-d5 (C₁₉H₃₁D₅O₃) provides a distinct isotopic signature (Δm/z +5) in LC-MS/MS MRM transitions, enabling precise calibration. This is critical in complex lipidomic studies, where co-eluting isomers (e.g., glycidyl stearate) complicate quantification .
Q. What experimental designs are recommended to study this compound degradation pathways?
Use kinetic modeling under controlled thermal stress (e.g., 170–250°C) with time-series sampling. Combine high-resolution MS (HRMS) for degradation product identification (e.g., glycidol, free palmitic acid) and isotopically labeled tracers (e.g., ¹³C-palmitate) to track ester cleavage. Statistical tools like ANOVA and principal component analysis (PCA) differentiate degradation pathways from artifact formation .
Q. Methodological Notes
- Standard Preparation : Dissolve this compound standards in methanol/2-propanol (1:1 v/v) to ensure stability. Store at -20°C under nitrogen to prevent epoxide ring hydrolysis .
- Data Interpretation : Compare GE profiles with fatty acid composition (e.g., GC-FID) to assess substrate specificity. For example, oleic acid-rich oils correlate with glycidyl oleate dominance .
- Quality Control : Include blanks and spiked recovery samples (70–120% recovery range) to validate method accuracy. Report limits of detection (LOD) and quantification (LOQ) based on signal-to-noise ratios .
Propriétés
IUPAC Name |
oxiran-2-ylmethyl hexadecanoate | |
---|---|---|
Source | PubChem | |
URL | https://pubchem.ncbi.nlm.nih.gov | |
Description | Data deposited in or computed by PubChem | |
InChI |
InChI=1S/C19H36O3/c1-2-3-4-5-6-7-8-9-10-11-12-13-14-15-19(20)22-17-18-16-21-18/h18H,2-17H2,1H3 | |
Source | PubChem | |
URL | https://pubchem.ncbi.nlm.nih.gov | |
Description | Data deposited in or computed by PubChem | |
InChI Key |
KYVUJPJYTYQNGJ-UHFFFAOYSA-N | |
Source | PubChem | |
URL | https://pubchem.ncbi.nlm.nih.gov | |
Description | Data deposited in or computed by PubChem | |
Canonical SMILES |
CCCCCCCCCCCCCCCC(=O)OCC1CO1 | |
Source | PubChem | |
URL | https://pubchem.ncbi.nlm.nih.gov | |
Description | Data deposited in or computed by PubChem | |
Molecular Formula |
C19H36O3 | |
Source | PubChem | |
URL | https://pubchem.ncbi.nlm.nih.gov | |
Description | Data deposited in or computed by PubChem | |
DSSTOX Substance ID |
DTXSID80324377 | |
Record name | Glycidyl Palmitate | |
Source | EPA DSSTox | |
URL | https://comptox.epa.gov/dashboard/DTXSID80324377 | |
Description | DSSTox provides a high quality public chemistry resource for supporting improved predictive toxicology. | |
Molecular Weight |
312.5 g/mol | |
Source | PubChem | |
URL | https://pubchem.ncbi.nlm.nih.gov | |
Description | Data deposited in or computed by PubChem | |
CAS No. |
7501-44-2 | |
Record name | NSC406558 | |
Source | DTP/NCI | |
URL | https://dtp.cancer.gov/dtpstandard/servlet/dwindex?searchtype=NSC&outputformat=html&searchlist=406558 | |
Description | The NCI Development Therapeutics Program (DTP) provides services and resources to the academic and private-sector research communities worldwide to facilitate the discovery and development of new cancer therapeutic agents. | |
Explanation | Unless otherwise indicated, all text within NCI products is free of copyright and may be reused without our permission. Credit the National Cancer Institute as the source. | |
Record name | Glycidyl Palmitate | |
Source | EPA DSSTox | |
URL | https://comptox.epa.gov/dashboard/DTXSID80324377 | |
Description | DSSTox provides a high quality public chemistry resource for supporting improved predictive toxicology. | |
Retrosynthesis Analysis
AI-Powered Synthesis Planning: Our tool employs the Template_relevance Pistachio, Template_relevance Bkms_metabolic, Template_relevance Pistachio_ringbreaker, Template_relevance Reaxys, Template_relevance Reaxys_biocatalysis model, leveraging a vast database of chemical reactions to predict feasible synthetic routes.
One-Step Synthesis Focus: Specifically designed for one-step synthesis, it provides concise and direct routes for your target compounds, streamlining the synthesis process.
Accurate Predictions: Utilizing the extensive PISTACHIO, BKMS_METABOLIC, PISTACHIO_RINGBREAKER, REAXYS, REAXYS_BIOCATALYSIS database, our tool offers high-accuracy predictions, reflecting the latest in chemical research and data.
Strategy Settings
Precursor scoring | Relevance Heuristic |
---|---|
Min. plausibility | 0.01 |
Model | Template_relevance |
Template Set | Pistachio/Bkms_metabolic/Pistachio_ringbreaker/Reaxys/Reaxys_biocatalysis |
Top-N result to add to graph | 6 |
Feasible Synthetic Routes
Avertissement et informations sur les produits de recherche in vitro
Veuillez noter que tous les articles et informations sur les produits présentés sur BenchChem sont destinés uniquement à des fins informatives. Les produits disponibles à l'achat sur BenchChem sont spécifiquement conçus pour des études in vitro, qui sont réalisées en dehors des organismes vivants. Les études in vitro, dérivées du terme latin "in verre", impliquent des expériences réalisées dans des environnements de laboratoire contrôlés à l'aide de cellules ou de tissus. Il est important de noter que ces produits ne sont pas classés comme médicaments et n'ont pas reçu l'approbation de la FDA pour la prévention, le traitement ou la guérison de toute condition médicale, affection ou maladie. Nous devons souligner que toute forme d'introduction corporelle de ces produits chez les humains ou les animaux est strictement interdite par la loi. Il est essentiel de respecter ces directives pour assurer la conformité aux normes légales et éthiques en matière de recherche et d'expérimentation.