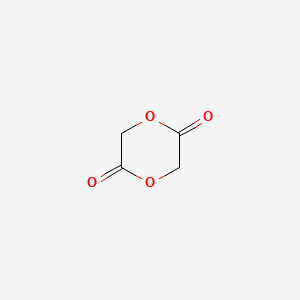
Glycolide
Vue d'ensemble
Description
ABH001 is under investigation in clinical trial NCT01749306 (A Study of the Efficacy and Safety of ABH001 in the Treatment of Patients With Epidermolysis Bullosa Who Have Wounds That Are Not Healing).
See also: Polyglactin 370 (monomer of); Polyglactin 910 (monomer of); Poliglecaprone 25 (monomer of) ... View More ...
Mécanisme D'action
Target of Action
Glycolide, also known as 1,4-Dioxane-2,5-dione, is a cyclic dimer of α-hydroxy acid . Its primary targets are the biological systems where it is used to form aliphatic polyesters . These polyesters are used in various medical applications, including the fabrication of artificial blood vessels .
Mode of Action
This compound interacts with its targets through a process known as ring-opening polymerization . This process involves the breaking of the cyclic structure of this compound, allowing it to form long-chain polymers . The resulting polymers are highly crystalline and biodegradable .
Biochemical Pathways
The primary biochemical pathway involved in the action of this compound is the polymerization pathway . This compound is synthesized by the dimerization of glycolic acid . The resulting polymers can be used to form poly(lactic-co-glycolide) (PGLA) via copolymerization with lactide monomer . PGLA has various applications, including use in bone repair applications and drug delivery .
Pharmacokinetics
The pharmacokinetics of this compound and its polymers are largely dependent on their physical and chemical properties. As a low toxic synthetic biodegradable polymer, it is majorly used in medical applications . .
Result of Action
The result of this compound’s action is the formation of biodegradable polymers that can be used in various medical applications . For example, porous PGLA microsphere scaffolds are used in bone repair applications . Encapsulated PGLA nanoparticles can be used in drug delivery and tissue engineering applications .
Action Environment
The action, efficacy, and stability of this compound and its polymers can be influenced by various environmental factors. For instance, the rate of biodegradation of the polymers can be affected by factors such as pH, temperature, and the presence of enzymes
Analyse Biochimique
Biochemical Properties
Glycolide plays a pivotal role in biochemical reactions, particularly in the synthesis of polyglycolic acid through ring-opening polymerization. This process involves the interaction of this compound with various catalysts and initiators, such as stannous octoate and tin(II) chloride, which facilitate the polymerization reaction . The resulting polyglycolic acid exhibits high tensile strength and remarkable hydrolytic stability, making it suitable for medical applications like absorbable sutures and drug delivery systems .
Cellular Effects
This compound and its derivatives, such as poly(lactic-co-glycolic acid) (PLGA), have been extensively studied for their effects on cellular processes. These compounds are known to influence cell function by enhancing cellular uptake and promoting controlled drug release. For instance, PLGA nanoparticles have been shown to improve the intracellular delivery of therapeutic agents, thereby enhancing their efficacy . This compound-based materials also impact cell signaling pathways and gene expression, contributing to their potential in cancer therapy and tissue engineering .
Molecular Mechanism
At the molecular level, this compound exerts its effects through various binding interactions with biomolecules. The ring-opening polymerization of this compound involves the formation of ester linkages, which contribute to the stability and mechanical properties of the resulting polymer . Additionally, this compound-based polymers can interact with enzymes and proteins, leading to enzyme inhibition or activation. These interactions play a crucial role in the biodegradation and biocompatibility of this compound-based materials .
Temporal Effects in Laboratory Settings
The stability and degradation of this compound in laboratory settings have been well-documented. This compound-based polymers, such as polyglycolic acid, exhibit a gradual decrease in weight and mechanical properties over time due to hydrolytic degradation . This degradation process is influenced by factors such as temperature and pH, with higher temperatures accelerating the degradation rate. Long-term studies have shown that this compound-based materials maintain their biocompatibility and functionality, making them suitable for various biomedical applications .
Dosage Effects in Animal Models
The effects of this compound vary with different dosages in animal models. Studies have demonstrated that low doses of this compound-based materials are well-tolerated and exhibit minimal toxicity . Higher doses can lead to adverse effects, such as inflammation and tissue damage. It is essential to determine the optimal dosage to maximize the therapeutic benefits while minimizing potential side effects . This compound’s biocompatibility and controlled degradation make it a promising candidate for drug delivery and tissue engineering applications.
Metabolic Pathways
This compound is involved in various metabolic pathways, primarily through its conversion to glycolic acid. Glycolic acid can be further metabolized by enzymes such as glycolate oxidase, leading to the production of oxalic acid or carbon dioxide . These metabolic pathways play a crucial role in the biodegradation and clearance of this compound-based materials from the body. The interaction of this compound with specific enzymes and cofactors influences the metabolic flux and overall efficacy of this compound-based therapies .
Transport and Distribution
The transport and distribution of this compound within cells and tissues are facilitated by various transporters and binding proteins. This compound-based nanoparticles, for instance, can be efficiently taken up by cells through endocytosis, leading to their accumulation in specific cellular compartments . The surface properties of these nanoparticles, such as charge and hydrophilicity, significantly influence their distribution and localization within tissues . Understanding these transport mechanisms is essential for optimizing the delivery and efficacy of this compound-based therapeutics.
Subcellular Localization
This compound and its derivatives exhibit specific subcellular localization patterns that influence their activity and function. For example, this compound-based nanoparticles can localize to lysosomes, where they undergo degradation and release their therapeutic payload . The subcellular localization of this compound is influenced by targeting signals and post-translational modifications, which direct the compound to specific compartments or organelles . These localization patterns are crucial for the effective delivery and action of this compound-based therapies.
Propriétés
IUPAC Name |
1,4-dioxane-2,5-dione | |
---|---|---|
Source | PubChem | |
URL | https://pubchem.ncbi.nlm.nih.gov | |
Description | Data deposited in or computed by PubChem | |
InChI |
InChI=1S/C4H4O4/c5-3-1-7-4(6)2-8-3/h1-2H2 | |
Source | PubChem | |
URL | https://pubchem.ncbi.nlm.nih.gov | |
Description | Data deposited in or computed by PubChem | |
InChI Key |
RKDVKSZUMVYZHH-UHFFFAOYSA-N | |
Source | PubChem | |
URL | https://pubchem.ncbi.nlm.nih.gov | |
Description | Data deposited in or computed by PubChem | |
Canonical SMILES |
C1C(=O)OCC(=O)O1 | |
Source | PubChem | |
URL | https://pubchem.ncbi.nlm.nih.gov | |
Description | Data deposited in or computed by PubChem | |
Molecular Formula |
C4H4O4 | |
Source | PubChem | |
URL | https://pubchem.ncbi.nlm.nih.gov | |
Description | Data deposited in or computed by PubChem | |
Related CAS |
26202-08-4 | |
Record name | Glycolide polymer | |
Source | CAS Common Chemistry | |
URL | https://commonchemistry.cas.org/detail?cas_rn=26202-08-4 | |
Description | CAS Common Chemistry is an open community resource for accessing chemical information. Nearly 500,000 chemical substances from CAS REGISTRY cover areas of community interest, including common and frequently regulated chemicals, and those relevant to high school and undergraduate chemistry classes. This chemical information, curated by our expert scientists, is provided in alignment with our mission as a division of the American Chemical Society. | |
Explanation | The data from CAS Common Chemistry is provided under a CC-BY-NC 4.0 license, unless otherwise stated. | |
DSSTOX Substance ID |
DTXSID8060115 | |
Record name | 1,4-Dioxane-2,5-dione | |
Source | EPA DSSTox | |
URL | https://comptox.epa.gov/dashboard/DTXSID8060115 | |
Description | DSSTox provides a high quality public chemistry resource for supporting improved predictive toxicology. | |
Molecular Weight |
116.07 g/mol | |
Source | PubChem | |
URL | https://pubchem.ncbi.nlm.nih.gov | |
Description | Data deposited in or computed by PubChem | |
CAS No. |
502-97-6 | |
Record name | Glycolide | |
Source | CAS Common Chemistry | |
URL | https://commonchemistry.cas.org/detail?cas_rn=502-97-6 | |
Description | CAS Common Chemistry is an open community resource for accessing chemical information. Nearly 500,000 chemical substances from CAS REGISTRY cover areas of community interest, including common and frequently regulated chemicals, and those relevant to high school and undergraduate chemistry classes. This chemical information, curated by our expert scientists, is provided in alignment with our mission as a division of the American Chemical Society. | |
Explanation | The data from CAS Common Chemistry is provided under a CC-BY-NC 4.0 license, unless otherwise stated. | |
Record name | Glycolide | |
Source | ChemIDplus | |
URL | https://pubchem.ncbi.nlm.nih.gov/substance/?source=chemidplus&sourceid=0000502976 | |
Description | ChemIDplus is a free, web search system that provides access to the structure and nomenclature authority files used for the identification of chemical substances cited in National Library of Medicine (NLM) databases, including the TOXNET system. | |
Record name | ABH001 | |
Source | DrugBank | |
URL | https://www.drugbank.ca/drugs/DB16177 | |
Description | The DrugBank database is a unique bioinformatics and cheminformatics resource that combines detailed drug (i.e. chemical, pharmacological and pharmaceutical) data with comprehensive drug target (i.e. sequence, structure, and pathway) information. | |
Explanation | Creative Common's Attribution-NonCommercial 4.0 International License (http://creativecommons.org/licenses/by-nc/4.0/legalcode) | |
Record name | Glycolide | |
Source | DTP/NCI | |
URL | https://dtp.cancer.gov/dtpstandard/servlet/dwindex?searchtype=NSC&outputformat=html&searchlist=403079 | |
Description | The NCI Development Therapeutics Program (DTP) provides services and resources to the academic and private-sector research communities worldwide to facilitate the discovery and development of new cancer therapeutic agents. | |
Explanation | Unless otherwise indicated, all text within NCI products is free of copyright and may be reused without our permission. Credit the National Cancer Institute as the source. | |
Record name | 1,4-Dioxane-2,5-dione | |
Source | EPA DSSTox | |
URL | https://comptox.epa.gov/dashboard/DTXSID8060115 | |
Description | DSSTox provides a high quality public chemistry resource for supporting improved predictive toxicology. | |
Record name | GLYCOLIDE | |
Source | FDA Global Substance Registration System (GSRS) | |
URL | https://gsrs.ncats.nih.gov/ginas/app/beta/substances/YRZ676PGU6 | |
Description | The FDA Global Substance Registration System (GSRS) enables the efficient and accurate exchange of information on what substances are in regulated products. Instead of relying on names, which vary across regulatory domains, countries, and regions, the GSRS knowledge base makes it possible for substances to be defined by standardized, scientific descriptions. | |
Explanation | Unless otherwise noted, the contents of the FDA website (www.fda.gov), both text and graphics, are not copyrighted. They are in the public domain and may be republished, reprinted and otherwise used freely by anyone without the need to obtain permission from FDA. Credit to the U.S. Food and Drug Administration as the source is appreciated but not required. | |
Synthesis routes and methods
Procedure details
Retrosynthesis Analysis
AI-Powered Synthesis Planning: Our tool employs the Template_relevance Pistachio, Template_relevance Bkms_metabolic, Template_relevance Pistachio_ringbreaker, Template_relevance Reaxys, Template_relevance Reaxys_biocatalysis model, leveraging a vast database of chemical reactions to predict feasible synthetic routes.
One-Step Synthesis Focus: Specifically designed for one-step synthesis, it provides concise and direct routes for your target compounds, streamlining the synthesis process.
Accurate Predictions: Utilizing the extensive PISTACHIO, BKMS_METABOLIC, PISTACHIO_RINGBREAKER, REAXYS, REAXYS_BIOCATALYSIS database, our tool offers high-accuracy predictions, reflecting the latest in chemical research and data.
Strategy Settings
Precursor scoring | Relevance Heuristic |
---|---|
Min. plausibility | 0.01 |
Model | Template_relevance |
Template Set | Pistachio/Bkms_metabolic/Pistachio_ringbreaker/Reaxys/Reaxys_biocatalysis |
Top-N result to add to graph | 6 |
Feasible Synthetic Routes
Q1: What is the molecular formula and weight of glycolide?
A1: this compound has the molecular formula C4H4O4 and a molecular weight of 116.07 g/mol.
Q2: Is there spectroscopic data available for this compound?
A2: Yes, researchers frequently utilize various spectroscopic techniques to characterize this compound-containing polymers. These techniques include: * Nuclear Magnetic Resonance (NMR) Spectroscopy: 1H and 13C NMR provide detailed information about the polymer's microstructure, including the arrangement of this compound units within the copolymer chain [, , ]. * Fourier Transform Infrared (FTIR) Spectroscopy: FTIR helps identify functional groups and analyze intermolecular interactions. For instance, FTIR analysis confirmed the absence of drug-polymer interactions in 5-fluorouracil-loaded microspheres composed of poly(this compound-co-ε-caprolactone) and ethylcellulose [].
Q3: What are the key properties that make this compound-based polymers suitable for biomedical applications?
A3: this compound-based polymers, primarily poly(lactic-co-glycolic acid) (PLGA), are favored in biomedicine due to their: * Biocompatibility: These polymers exhibit excellent compatibility with biological systems, eliciting minimal adverse reactions [, ]. * Biodegradability: PLGA degrades into naturally occurring, non-toxic metabolites (lactic acid and glycolic acid) that are readily metabolized by the body [, , ]. * Tunable Degradation Rates: By adjusting the ratio of this compound to other monomers like lactide, researchers can tailor the degradation rate of the copolymer, allowing for controlled drug release over desired timeframes [, , , ].
Q4: How does the presence of this compound affect the properties of its copolymers?
A4: The incorporation of this compound significantly influences the properties of copolymers: * Hydrolytic Degradation: this compound units enhance the hydrophilicity of the copolymer, leading to faster hydrolytic degradation rates compared to polymers without this compound [, , ]. * Thermal and Mechanical Properties: this compound content impacts the glass transition temperature (Tg), crystallinity, and mechanical strength of copolymers, making them suitable for various applications like sutures and scaffolds [, , ].
Q5: What are some specific examples of how this compound-based materials are being used in biomedical applications?
A5: this compound-based materials, particularly PLGA, have found widespread use in various biomedical applications, including: * Drug Delivery Systems: PLGA microspheres and nanoparticles enable controlled release of various therapeutic agents, including anticancer drugs [, , ], antipsychotics [], and ophthalmic drugs []. * Tissue Engineering Scaffolds: Porous scaffolds fabricated from PLGA and its copolymers support cell growth and tissue regeneration in applications like bone tissue engineering [, , ]. * Surgical Sutures: Biodegradable sutures made from this compound-containing polymers provide temporary wound support and eliminate the need for suture removal [, ].
Q6: How is this compound typically polymerized to form copolymers?
A6: this compound commonly undergoes ring-opening polymerization (ROP) to form copolymers. This process involves: * Initiators: Various initiators, such as stannous octoate [, ], zirconium acetylacetonate [], lithium, and magnesium compounds [], facilitate the ring-opening of this compound and initiate the polymerization process. * Copolymerization: this compound is copolymerized with other monomers like lactide [, , , ], ε-caprolactone [, , ], and trimethylene carbonate [] to achieve desired properties.
Q7: What is the significance of transesterification reactions during the polymerization of this compound?
A7: Transesterification reactions, occurring during polymerization, significantly influence the microstructure of this compound copolymers [, ]. They can lead to: * Randomization of Monomer Units: Affecting the distribution of different monomer units along the polymer chain, impacting its properties [, ]. * Control over Microstructure: By manipulating reaction conditions like temperature and catalyst type, researchers can control the extent of transesterification and, consequently, the copolymer's microstructure and properties [, ].
Q8: How does the stability of this compound-based materials vary under different conditions?
A8: The stability of this compound-based materials is influenced by factors such as: * pH: this compound-containing polymers exhibit enhanced degradation in alkaline media due to the hydrolysis of ester bonds []. * Temperature: Elevated temperatures can accelerate the degradation process [, ]. * Sterilization Methods: Sterilization techniques like gamma irradiation can impact the material's properties, while electron beam sterilization shows less impact [].
Q9: What strategies can be employed to improve the stability or tailor the degradation of this compound-based formulations?
A9: Several strategies are used to modulate the stability and degradation of these formulations: * End-capping: Modifying the terminal groups of the polymer chains can enhance stability, as seen with end-capped polythis compound–caprolactone copolymers exhibiting controlled hydrolytic degradation []. * Monomer Ratio: Adjusting the ratio of this compound to other monomers in the copolymer influences its overall hydrophilicity and degradation rate [, , ]. * Additives: Incorporating additives like polyethers can enhance the hydrophilicity of the material and potentially influence its degradation profile [].
Q10: What analytical techniques are commonly employed to characterize and quantify this compound and its copolymers?
A10: Researchers utilize various analytical techniques for characterizing this compound-containing polymers, including: * Gel Permeation Chromatography (GPC): This technique determines the molecular weight and polydispersity of the synthesized polymers [, ]. * Differential Scanning Calorimetry (DSC): DSC analyzes the thermal properties of the polymers, such as their glass transition temperature and melting behavior, providing insights into their morphology and potential applications [, , ]. * X-ray Diffraction (XRD): XRD helps determine the crystallinity and crystal structure of this compound-containing polymers, which can be related to their mechanical properties and degradation behavior [, ].
Avertissement et informations sur les produits de recherche in vitro
Veuillez noter que tous les articles et informations sur les produits présentés sur BenchChem sont destinés uniquement à des fins informatives. Les produits disponibles à l'achat sur BenchChem sont spécifiquement conçus pour des études in vitro, qui sont réalisées en dehors des organismes vivants. Les études in vitro, dérivées du terme latin "in verre", impliquent des expériences réalisées dans des environnements de laboratoire contrôlés à l'aide de cellules ou de tissus. Il est important de noter que ces produits ne sont pas classés comme médicaments et n'ont pas reçu l'approbation de la FDA pour la prévention, le traitement ou la guérison de toute condition médicale, affection ou maladie. Nous devons souligner que toute forme d'introduction corporelle de ces produits chez les humains ou les animaux est strictement interdite par la loi. Il est essentiel de respecter ces directives pour assurer la conformité aux normes légales et éthiques en matière de recherche et d'expérimentation.