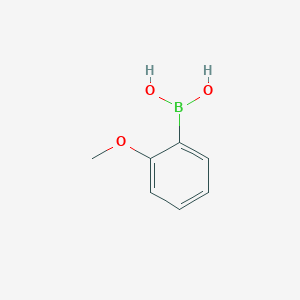
Acide 2-méthoxyphénylboronique
Vue d'ensemble
Description
2-Methoxyphenylboronic acid, also known as (2-Methoxyphenyl)boric acid, is an organoboron compound with the molecular formula CH₃OC₆H₄B(OH)₂. This compound is a derivative of phenylboronic acid, where a methoxy group is substituted at the ortho position of the phenyl ring. It is widely used in organic synthesis, particularly in Suzuki-Miyaura cross-coupling reactions, due to its ability to form carbon-carbon bonds efficiently .
Applications De Recherche Scientifique
2-Methoxyphenylboronic acid has a wide range of applications in scientific research:
Chemistry: It is extensively used in the synthesis of complex organic molecules through cross-coupling reactions.
Biology: The compound is used in the development of boron-containing drugs and as a tool for studying boron metabolism in plants.
Mécanisme D'action
Target of Action
2-Methoxyphenylboronic acid is primarily used in the Suzuki–Miyaura (SM) cross-coupling reaction . The primary target of this compound is the palladium catalyst used in the SM reaction .
Mode of Action
In the SM cross-coupling reaction, 2-Methoxyphenylboronic acid acts as a nucleophile . The reaction involves two key steps: oxidative addition and transmetalation . In the oxidative addition step, the palladium catalyst forms a bond with an electrophilic organic group . In the transmetalation step, the 2-Methoxyphenylboronic acid is transferred from boron to palladium .
Biochemical Pathways
The SM cross-coupling reaction is a key biochemical pathway involving 2-Methoxyphenylboronic acid . This reaction is widely used for carbon–carbon bond formation . The success of this reaction is due to its mild and functional group tolerant conditions, and the use of a stable, readily prepared, and environmentally benign organoboron reagent .
Result of Action
The primary result of the action of 2-Methoxyphenylboronic acid in the SM cross-coupling reaction is the formation of a new carbon–carbon bond . This is a crucial step in the synthesis of many organic compounds .
Action Environment
The efficacy and stability of 2-Methoxyphenylboronic acid in the SM cross-coupling reaction can be influenced by various environmental factors. These include the presence of other reagents, the temperature, and the pH of the reaction environment . .
Analyse Biochimique
Biochemical Properties
It is known that boronic acids, including 2-Methoxyphenylboronic acid, play a crucial role in biochemical reactions, particularly in the Suzuki–Miyaura cross-coupling reaction . This reaction involves the coupling of an organoboron compound with an organic halide, catalyzed by a palladium complex .
Cellular Effects
A study has shown that halogenated phenylboronic acids, which are structurally similar to 2-Methoxyphenylboronic acid, exhibit antibacterial and antibiofilm activity against certain bacterial species .
Molecular Mechanism
The molecular mechanism of 2-Methoxyphenylboronic acid primarily involves its role in the Suzuki–Miyaura cross-coupling reaction . In this reaction, the boronic acid acts as a nucleophile, transferring an organic group to a palladium complex .
Temporal Effects in Laboratory Settings
It is known that the compound is stable under normal conditions .
Metabolic Pathways
Boronic acids are known to be involved in various biochemical reactions, including the Suzuki–Miyaura cross-coupling reaction .
Méthodes De Préparation
Synthetic Routes and Reaction Conditions: 2-Methoxyphenylboronic acid can be synthesized through several methods. One common approach involves the reaction of 2-methoxyphenylmagnesium bromide with trimethyl borate, followed by hydrolysis. The reaction conditions typically involve:
Reagents: 2-methoxyphenylmagnesium bromide, trimethyl borate
Solvent: Anhydrous ether
Temperature: Room temperature
Hydrolysis: Acidic work-up with hydrochloric acid
Industrial Production Methods: In industrial settings, the production of 2-methoxyphenylboronic acid often involves large-scale Grignard reactions, followed by purification through recrystallization. The process is optimized for high yield and purity, ensuring the compound meets the required specifications for various applications .
Analyse Des Réactions Chimiques
2-Methoxyphenylboronic acid undergoes several types of chemical reactions, including:
Suzuki-Miyaura Coupling: This reaction involves the coupling of 2-methoxyphenylboronic acid with aryl halides in the presence of a palladium catalyst and a base. The major product is a biaryl compound.
Oxidation: The compound can be oxidized to form 2-methoxybenzoic acid using oxidizing agents like hydrogen peroxide.
Substitution: It can undergo electrophilic aromatic substitution reactions, where the methoxy group directs the incoming electrophile to the ortho and para positions.
Common Reagents and Conditions:
Catalysts: Palladium complexes for coupling reactions
Bases: Potassium carbonate, sodium hydroxide
Solvents: Tetrahydrofuran, ethanol
Temperature: Typically between 50-100°C
Major Products:
Biaryl Compounds: From Suzuki-Miyaura coupling
2-Methoxybenzoic Acid: From oxidation reactions
Comparaison Avec Des Composés Similaires
2-Methoxyphenylboronic acid can be compared with other boronic acids, such as:
Phenylboronic Acid: Lacks the methoxy group, making it less electron-rich and less reactive in certain coupling reactions.
4-Methoxyphenylboronic Acid: The methoxy group is at the para position, which affects the electronic properties and reactivity differently.
2-Fluorophenylboronic Acid: The fluorine substituent provides different steric and electronic effects compared to the methoxy group.
The unique positioning of the methoxy group in 2-methoxyphenylboronic acid enhances its reactivity and selectivity in various chemical reactions .
Activité Biologique
2-Methoxyphenylboronic acid (CAS Number: 5720-06-9) is a significant compound in medicinal chemistry, particularly noted for its biological activities. This article explores its antibacterial properties, potential applications in drug development, and the underlying mechanisms of action.
- Molecular Formula : C₇H₉BO₃
- Molecular Weight : 151.96 g/mol
- Density : 1.2 g/cm³
- Melting Point : 105-110 °C
- Boiling Point : 306.8 °C at 760 mmHg
Antibacterial Activity
Recent studies have highlighted the antibacterial and antibiofilm activities of various boronic acids, including 2-methoxyphenylboronic acid. Research indicates that compounds like 3,5-diiodo-2-methoxyphenylboronic acid (DIMPBA) exhibit significant antibacterial effects against Vibrio species, which are known to cause foodborne illnesses.
Minimum Inhibitory Concentrations (MIC)
The following table summarizes the MIC values for selected boronic acids against Vibrio parahaemolyticus and Vibrio harveyi:
Compound Name | MIC (µg/mL) | Growth Inhibition (%) |
---|---|---|
2-Methoxyphenylboronic acid | >500 | Not specified |
3,5-Diiodo-2-methoxyphenylboronic acid (DIMPBA) | 100 | 30.3 |
2-Fluoro-5-iodophenylboronic acid (FIPBA) | 100 | 24.1 |
Control (No treatment) | >500 | - |
DIMPBA has shown a remarkable ability to inhibit biofilm formation, which is crucial in preventing chronic infections associated with biofilms.
The mechanism by which boronic acids exert their antibacterial effects is primarily through the inhibition of bacterial virulence factors. DIMPBA and similar compounds have been shown to reduce motility, hydrophobicity, and indole synthesis in bacteria, thereby hampering their ability to form biofilms and establish infections .
Case Studies
- Antibiofilm Properties : A study evaluating the efficacy of DIMPBA against biofilms formed by Vibrio parahaemolyticus revealed that at a concentration of 100 µg/mL, it significantly reduced biofilm biomass and inhibited virulence factor expression .
- Pharmacological Characterization : In another investigation, 2-methoxyphenylboronic acid was used as a substrate in Suzuki coupling reactions, demonstrating its utility in synthesizing more complex pharmaceutical compounds . This highlights its role not only as a therapeutic agent but also as a building block in drug development.
Applications in Drug Development
The unique properties of boronic acids make them valuable in various fields:
- Antimicrobial Agents : Their ability to combat resistant bacterial strains positions them as critical candidates for new antibiotics.
- Cancer Therapy : Boronic acids are being explored for their potential in targeting specific cancer pathways due to their ability to interact with biological molecules selectively .
Propriétés
IUPAC Name |
(2-methoxyphenyl)boronic acid | |
---|---|---|
Source | PubChem | |
URL | https://pubchem.ncbi.nlm.nih.gov | |
Description | Data deposited in or computed by PubChem | |
InChI |
InChI=1S/C7H9BO3/c1-11-7-5-3-2-4-6(7)8(9)10/h2-5,9-10H,1H3 | |
Source | PubChem | |
URL | https://pubchem.ncbi.nlm.nih.gov | |
Description | Data deposited in or computed by PubChem | |
InChI Key |
ROEQGIFOWRQYHD-UHFFFAOYSA-N | |
Source | PubChem | |
URL | https://pubchem.ncbi.nlm.nih.gov | |
Description | Data deposited in or computed by PubChem | |
Canonical SMILES |
B(C1=CC=CC=C1OC)(O)O | |
Source | PubChem | |
URL | https://pubchem.ncbi.nlm.nih.gov | |
Description | Data deposited in or computed by PubChem | |
Molecular Formula |
C7H9BO3 | |
Source | PubChem | |
URL | https://pubchem.ncbi.nlm.nih.gov | |
Description | Data deposited in or computed by PubChem | |
DSSTOX Substance ID |
DTXSID40370057 | |
Record name | 2-Methoxyphenylboronic acid | |
Source | EPA DSSTox | |
URL | https://comptox.epa.gov/dashboard/DTXSID40370057 | |
Description | DSSTox provides a high quality public chemistry resource for supporting improved predictive toxicology. | |
Molecular Weight |
151.96 g/mol | |
Source | PubChem | |
URL | https://pubchem.ncbi.nlm.nih.gov | |
Description | Data deposited in or computed by PubChem | |
CAS No. |
5720-06-9 | |
Record name | 2-Methoxyphenylboronic acid | |
Source | CAS Common Chemistry | |
URL | https://commonchemistry.cas.org/detail?cas_rn=5720-06-9 | |
Description | CAS Common Chemistry is an open community resource for accessing chemical information. Nearly 500,000 chemical substances from CAS REGISTRY cover areas of community interest, including common and frequently regulated chemicals, and those relevant to high school and undergraduate chemistry classes. This chemical information, curated by our expert scientists, is provided in alignment with our mission as a division of the American Chemical Society. | |
Explanation | The data from CAS Common Chemistry is provided under a CC-BY-NC 4.0 license, unless otherwise stated. | |
Record name | 2-Methoxyphenylboronic acid | |
Source | EPA DSSTox | |
URL | https://comptox.epa.gov/dashboard/DTXSID40370057 | |
Description | DSSTox provides a high quality public chemistry resource for supporting improved predictive toxicology. | |
Synthesis routes and methods I
Procedure details
Synthesis routes and methods II
Procedure details
Synthesis routes and methods III
Procedure details
Synthesis routes and methods IV
Procedure details
Retrosynthesis Analysis
AI-Powered Synthesis Planning: Our tool employs the Template_relevance Pistachio, Template_relevance Bkms_metabolic, Template_relevance Pistachio_ringbreaker, Template_relevance Reaxys, Template_relevance Reaxys_biocatalysis model, leveraging a vast database of chemical reactions to predict feasible synthetic routes.
One-Step Synthesis Focus: Specifically designed for one-step synthesis, it provides concise and direct routes for your target compounds, streamlining the synthesis process.
Accurate Predictions: Utilizing the extensive PISTACHIO, BKMS_METABOLIC, PISTACHIO_RINGBREAKER, REAXYS, REAXYS_BIOCATALYSIS database, our tool offers high-accuracy predictions, reflecting the latest in chemical research and data.
Strategy Settings
Precursor scoring | Relevance Heuristic |
---|---|
Min. plausibility | 0.01 |
Model | Template_relevance |
Template Set | Pistachio/Bkms_metabolic/Pistachio_ringbreaker/Reaxys/Reaxys_biocatalysis |
Top-N result to add to graph | 6 |
Feasible Synthetic Routes
Q1: What is the primary use of 2-Methoxyphenylboronic acid in organic synthesis?
A: 2-Methoxyphenylboronic acid serves as a crucial building block in Suzuki-Miyaura coupling reactions. [, , , ] This palladium-catalyzed reaction enables the formation of carbon-carbon bonds between 2-Methoxyphenylboronic acid and various organic halides, providing a versatile route to synthesize complex molecules. For example, it plays a key role in synthesizing substituted Benzo[c]chromen-6-ones through a Suzuki coupling and lactonization sequence. []
Q2: Are there any studies exploring the biological activity of compounds derived from 2-Methoxyphenylboronic acid?
A: Yes, derivatives of 2-Methoxyphenylboronic acid, particularly halogenated analogs, have shown promising antibacterial and antibiofilm properties. For instance, 3,5-diiodo-2-methoxyphenylboronic acid (DIMPBA) displayed potent activity against Vibrio parahaemolyticus and Vibrio harveyi, inhibiting both planktonic growth and biofilm formation. [] These findings suggest potential applications for these compounds in addressing challenges related to bacterial infections, particularly in aquaculture and food safety.
Q3: What are the advantages of using ionic liquids in Suzuki coupling reactions involving 2-Methoxyphenylboronic acid?
A: Research indicates that incorporating ionic liquids, such as [BMIM][PF6], can significantly accelerate Suzuki coupling reactions involving 2-Methoxyphenylboronic acid. [] Moreover, employing Lewis acidic ionic liquids like [BMIM][Al2Cl7] or [TMAH][Al2Cl7] allows for a one-pot synthesis of benzo[c]chromen-6-ones, streamlining what would traditionally be a multi-step process. [] This highlights the potential of ionic liquids to improve reaction efficiency and simplify synthetic procedures.
Q4: Is there any structural information available for 2-Methoxyphenylboronic acid or its derivatives?
A: Yes, X-ray crystallographic analysis of 2-(2-Hydroxyphenyl)-1-azaazulene, a derivative of 2-Methoxyphenylboronic acid, revealed a planar ring system stabilized by an intramolecular hydrogen bond between the phenolic hydrogen atom and the nitrogen atom of the azaazulenyl ring. [] This information provides valuable insights into the molecular geometry and potential intermolecular interactions of this class of compounds.
Q5: What analytical techniques are commonly employed to characterize 2-Methoxyphenylboronic acid and its derivatives?
A: Common analytical techniques used for characterizing 2-Methoxyphenylboronic acid and its derivatives include nuclear magnetic resonance (NMR) spectroscopy and infrared (IR) spectroscopy. [] For instance, the structure of 5-Fluoro-2-methoxyphenylboronic acid, synthesized from 2-Methoxyphenylboronic acid, was confirmed using 1H NMR and IR. [] These techniques help to elucidate the structure and purity of the synthesized compounds.
Avertissement et informations sur les produits de recherche in vitro
Veuillez noter que tous les articles et informations sur les produits présentés sur BenchChem sont destinés uniquement à des fins informatives. Les produits disponibles à l'achat sur BenchChem sont spécifiquement conçus pour des études in vitro, qui sont réalisées en dehors des organismes vivants. Les études in vitro, dérivées du terme latin "in verre", impliquent des expériences réalisées dans des environnements de laboratoire contrôlés à l'aide de cellules ou de tissus. Il est important de noter que ces produits ne sont pas classés comme médicaments et n'ont pas reçu l'approbation de la FDA pour la prévention, le traitement ou la guérison de toute condition médicale, affection ou maladie. Nous devons souligner que toute forme d'introduction corporelle de ces produits chez les humains ou les animaux est strictement interdite par la loi. Il est essentiel de respecter ces directives pour assurer la conformité aux normes légales et éthiques en matière de recherche et d'expérimentation.