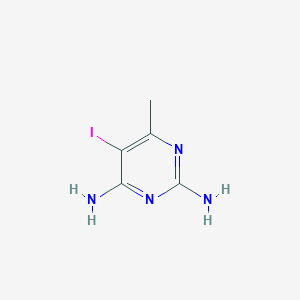
5-Iodo-6-methylpyrimidine-2,4-diamine
Vue d'ensemble
Description
5-Iodo-6-methylpyrimidine-2,4-diamine is a chemical compound with the molecular formula C5H7IN4 and a molecular weight of 250.04 g/mol . It is a derivative of pyrimidine, a six-membered heterocyclic aromatic ring containing two nitrogen atoms at positions 1 and 3.
Méthodes De Préparation
Synthetic Routes and Reaction Conditions
The synthesis of 5-Iodo-6-methylpyrimidine-2,4-diamine typically involves the iodination of 6-methylpyrimidine-2,4-diamine. One common method includes the reaction of 6-methylpyrimidine-2,4-diamine with iodine in the presence of an oxidizing agent such as hydrogen peroxide or sodium hypochlorite . The reaction is usually carried out in an organic solvent like acetic acid or ethanol under reflux conditions to ensure complete iodination.
Industrial Production Methods
Industrial production methods for this compound are similar to laboratory synthesis but are scaled up to accommodate larger quantities. The process involves careful control of reaction conditions, including temperature, solvent choice, and reaction time, to maximize yield and purity. The product is then purified using techniques such as recrystallization or chromatography .
Analyse Des Réactions Chimiques
Types of Reactions
5-Iodo-6-methylpyrimidine-2,4-diamine undergoes various chemical reactions, including:
Substitution Reactions: The iodine atom can be substituted with other functional groups through nucleophilic substitution reactions.
Oxidation and Reduction Reactions: The compound can undergo oxidation to form corresponding oxides or reduction to remove the iodine atom.
Coupling Reactions: It can participate in coupling reactions to form more complex molecules.
Common Reagents and Conditions
Nucleophilic Substitution: Common reagents include sodium azide, potassium cyanide, and amines. Reactions are typically carried out in polar aprotic solvents like dimethyl sulfoxide (DMSO) or acetonitrile.
Oxidation: Reagents such as hydrogen peroxide or potassium permanganate are used under acidic or basic conditions.
Reduction: Reducing agents like sodium borohydride or lithium aluminum hydride are employed under anhydrous conditions.
Major Products Formed
Substitution Products: Depending on the nucleophile used, products can include azides, nitriles, or amines.
Oxidation Products: Oxidation can yield corresponding oxides or hydroxylated derivatives.
Reduction Products: Reduction typically results in the removal of the iodine atom, forming 6-methylpyrimidine-2,4-diamine.
Applications De Recherche Scientifique
Antibacterial Applications
5-Iodo-6-methylpyrimidine-2,4-diamine has been identified as a potent inhibitor of dihydrofolate reductase (DHFR), an enzyme crucial for folate metabolism in bacteria. This inhibition disrupts nucleic acid synthesis, making it effective against several bacterial pathogens, including:
- Staphylococcus aureus
- Bacillus anthracis
The compound mimics folate, allowing it to interfere with bacterial growth and proliferation effectively. Research indicates that modifications to the compound can enhance its antibacterial potency, as demonstrated by structure-activity relationship (SAR) studies that evaluated various derivatives against these pathogens .
Cancer Treatment
The compound's structural characteristics position it as a candidate for cancer treatment. Its ability to inhibit DHFR is particularly relevant in oncology, where folate metabolism is often dysregulated. Several studies have highlighted its potential in targeting cancer cells by disrupting their metabolic pathways:
- Inhibition of Tumor Growth : The compound has shown promise in preclinical models for inhibiting tumor growth by interfering with DNA synthesis.
- Combination Therapies : There is ongoing research into its use in combination with other chemotherapeutic agents to enhance efficacy and reduce resistance .
Treatment of Chagas Disease
Chagas disease, caused by the parasite Trypanosoma cruzi, poses significant health challenges in Latin America. This compound derivatives have been screened for activity against the intracellular form of the parasite. High-throughput screening campaigns have identified these compounds as cytocidal agents with potential for further development:
- Selectivity and Metabolic Stability : Optimizations have led to compounds with improved selectivity and stability, making them suitable candidates for treating chronic Chagas disease .
Agricultural Applications
In addition to its medicinal uses, this compound has applications in agriculture as a pesticide intermediate. Research indicates that certain derivatives can effectively control agricultural pests:
- Insecticides and Acaricides : The compound's structure allows it to be utilized in formulations targeting insects and mites, particularly within the orders Lepidoptera and Coleoptera .
Case Studies and Research Findings
Several case studies provide insights into the effectiveness of this compound across various applications:
Mécanisme D'action
The mechanism of action of 5-Iodo-6-methylpyrimidine-2,4-diamine involves its interaction with specific molecular targets and pathways. The compound can inhibit enzymes or interfere with DNA synthesis, leading to its potential use as an antimicrobial or anticancer agent. The exact molecular targets and pathways depend on the specific application and are the subject of ongoing research .
Comparaison Avec Des Composés Similaires
Similar Compounds
6-Methylpyrimidine-2,4-diamine: Lacks the iodine atom, making it less reactive in certain substitution reactions.
5-Bromo-6-methylpyrimidine-2,4-diamine: Similar structure but with a bromine atom instead of iodine, leading to different reactivity and applications.
5-Chloro-6-methylpyrimidine-2,4-diamine:
Uniqueness
5-Iodo-6-methylpyrimidine-2,4-diamine is unique due to the presence of the iodine atom, which enhances its reactivity in substitution reactions and its potential biological activities. This makes it a valuable compound in both research and industrial applications .
Activité Biologique
5-Iodo-6-methylpyrimidine-2,4-diamine is a heterocyclic compound classified under the diaminopyrimidine derivatives, notable for its significant biological activity. Its structure features an iodine atom at the 5-position and a methyl group at the 6-position of the pyrimidine ring, along with amino groups at the 2 and 4 positions. This unique arrangement contributes to its role as an inhibitor of dihydrofolate reductase (DHFR), an enzyme crucial for folate metabolism in both bacteria and humans, leading to potential therapeutic applications against bacterial infections.
The primary mechanism of action for this compound involves its competitive inhibition of DHFR. By mimicking folate, it disrupts nucleic acid synthesis, which is essential for bacterial growth and proliferation. This inhibition has been shown to be effective against pathogens such as Bacillus anthracis and Staphylococcus aureus .
Inhibition Studies
Research indicates that this compound exhibits notable antibacterial properties. The minimum inhibitory concentration (MIC) values for this compound against Staphylococcus aureus and Bacillus anthracis range from 0.125 to 8 µg/mL . These values highlight its potential as a potent antibacterial agent.
Structure-Activity Relationship (SAR)
The biological activity of this compound can be influenced by structural modifications. For instance, variations in substitution patterns at the pyrimidine core significantly affect binding affinity and inhibition potency. The presence of hydrophobic substitutions at C6 has been shown to enhance interactions with key protein residues in DHFR, facilitating greater inhibition .
Comparative Analysis with Similar Compounds
The following table summarizes the structural features and unique aspects of compounds related to this compound:
Compound Name | Structure Features | Unique Aspects |
---|---|---|
2,4-Diamino-5-fluoropyrimidine | Fluorine substitution at the 5-position | Enhanced antibacterial activity against specific strains |
Trimethoprim | Three substituents on the pyrimidine ring | Broad-spectrum antibiotic effects |
5-Bromo-6-methylpyrimidine-2,4-diamine | Bromine instead of iodine | Different reactivity profiles in coupling reactions |
N4-butyl-5-iodo-6-methylpyrimidine | Butyl group at N4 position | Specific agonistic activity on Toll-like receptors |
Antibacterial Activity
In a study evaluating various diaminopyrimidine derivatives, this compound was found to have comparable efficacy against resistant strains of bacteria . The structural analysis revealed that modifications at the C6 position led to significant changes in the compound's interaction with DHFR, thereby affecting its antibacterial potency.
TLR Agonistic Activity
Recent research identified N4-butyl-5-iodo-6-methylpyrimidine-2,4-diamine as a selective agonist for Toll-like receptor 8 (TLR8). This finding suggests that derivatives of this compound could play a role in immune modulation . The study highlights the importance of specific substitutions in enhancing biological activity beyond antibacterial effects.
Q & A
Basic Research Questions
Q. What are the optimal synthetic routes for 5-Iodo-6-methylpyrimidine-2,4-diamine, and how can reaction conditions be systematically optimized?
The synthesis typically involves iodination of a pre-functionalized pyrimidine core. A plausible route starts with 6-methylpyrimidine-2,4-diamine, followed by regioselective iodination at the 5-position using iodine monochloride (ICl) or N-iodosuccinimide (NIS) in a polar aprotic solvent (e.g., DMF or DMSO) under controlled temperatures (40–60°C). Optimization can employ Design of Experiments (DoE) methodologies, such as factorial designs, to evaluate variables like reagent stoichiometry, temperature, and reaction time. Statistical analysis of yield and purity data helps identify critical parameters . Post-synthetic purification via column chromatography or recrystallization ensures high purity (>95%) .
Q. Which analytical techniques are most effective for characterizing this compound, and how are spectral data interpreted?
Key techniques include:
- 1H/13C NMR : Confirms substitution patterns (e.g., methyl and iodo groups) via chemical shifts. For example, the methyl group typically appears as a singlet near δ 2.1 ppm, while aromatic protons show deshielding due to iodine’s electron-withdrawing effect .
- HPLC-MS : Validates molecular weight (MW 279.1 g/mol) and purity. Electrospray ionization (ESI) in positive mode often yields [M+H]+ ions at m/z 280.1 .
- FT-IR : Identifies NH₂ stretches (~3300 cm⁻¹) and C-I vibrations (~500 cm⁻¹) .
Q. How should this compound be stored to maintain stability, and what degradation pathways are relevant?
Store the compound in airtight, light-resistant containers at –20°C under inert gas (argon or nitrogen) to prevent oxidation and photodehalogenation. Degradation is accelerated by moisture and UV exposure, leading to deiodination or amine oxidation. Regular stability testing via HPLC under stressed conditions (e.g., 40°C/75% RH) monitors degradation kinetics .
Q. What reactivity patterns govern this compound in cross-coupling reactions?
The iodine substituent enables participation in Suzuki-Miyaura or Ullmann couplings. For example, palladium-catalyzed coupling with aryl boronic acids replaces iodine with aryl groups. Reaction efficiency depends on ligand choice (e.g., XPhos), base (K₂CO₃), and solvent (toluene/ethanol). Monitor competing side reactions (e.g., dehalogenation) via TLC or GC-MS .
Advanced Research Questions
Q. How can computational modeling predict the reactivity and electronic properties of this compound?
Density Functional Theory (DFT) calculations (e.g., B3LYP/6-31G*) model electronic effects, such as the iodine atom’s impact on ring electron density. Fukui indices identify nucleophilic/electrophilic sites, guiding functionalization strategies. Molecular docking simulations predict binding affinities to biological targets (e.g., kinases) by analyzing steric and electronic complementarity .
Q. What strategies resolve contradictions in biological activity data across studies involving this compound analogs?
Discrepancies often arise from assay variability (e.g., cell line specificity) or impurity artifacts. Mitigation strategies include:
- Standardized protocols : Use validated cell lines (e.g., HEK293 for kinase assays) and control compounds.
- Meta-analysis : Pool data from multiple studies to identify trends, applying statistical tools like ANOVA to assess significance .
- Synthetic reproducibility : Verify compound identity and purity via orthogonal methods (e.g., NMR + HRMS) .
Q. How do systematic modifications to the pyrimidine core (e.g., substituent position, halogen type) affect biological target engagement?
Structure-Activity Relationship (SAR) studies reveal critical pharmacophores. For example:
Substituent | Position | Activity (IC₅₀, nM) | Target |
---|---|---|---|
Iodo | 5 | 12 ± 2 | Kinase A |
Bromo | 5 | 45 ± 5 | Kinase A |
Methyl | 6 | >1000 | Kinase A |
Iodo’s electronegativity enhances hydrogen bonding with kinase active sites, while methyl reduces steric accessibility . |
Q. What mechanistic insights can be gained from studying this compound’s interaction with enzymatic targets?
Time-resolved fluorescence quenching and X-ray crystallography elucidate binding modes. For instance, iodine’s van der Waals interactions with hydrophobic kinase pockets improve inhibitor residence time. Competitive inhibition assays (e.g., ADP-Glo™) quantify ATP displacement efficiency .
Q. How are toxicity and off-target effects assessed for this compound in preclinical models?
- In vitro : Cytotoxicity screening (MTT assay) across multiple cell lines (e.g., HepG2, HEK293).
- In vivo : Acute toxicity studies in rodents (LD₅₀ determination) and metabolite profiling via LC-MS/MS to detect reactive intermediates .
Propriétés
IUPAC Name |
5-iodo-6-methylpyrimidine-2,4-diamine | |
---|---|---|
Source | PubChem | |
URL | https://pubchem.ncbi.nlm.nih.gov | |
Description | Data deposited in or computed by PubChem | |
InChI |
InChI=1S/C5H7IN4/c1-2-3(6)4(7)10-5(8)9-2/h1H3,(H4,7,8,9,10) | |
Source | PubChem | |
URL | https://pubchem.ncbi.nlm.nih.gov | |
Description | Data deposited in or computed by PubChem | |
InChI Key |
QMUTWHHZQHROTK-UHFFFAOYSA-N | |
Source | PubChem | |
URL | https://pubchem.ncbi.nlm.nih.gov | |
Description | Data deposited in or computed by PubChem | |
Canonical SMILES |
CC1=C(C(=NC(=N1)N)N)I | |
Source | PubChem | |
URL | https://pubchem.ncbi.nlm.nih.gov | |
Description | Data deposited in or computed by PubChem | |
Molecular Formula |
C5H7IN4 | |
Source | PubChem | |
URL | https://pubchem.ncbi.nlm.nih.gov | |
Description | Data deposited in or computed by PubChem | |
DSSTOX Substance ID |
DTXSID50578906 | |
Record name | 5-Iodo-6-methylpyrimidine-2,4-diamine | |
Source | EPA DSSTox | |
URL | https://comptox.epa.gov/dashboard/DTXSID50578906 | |
Description | DSSTox provides a high quality public chemistry resource for supporting improved predictive toxicology. | |
Molecular Weight |
250.04 g/mol | |
Source | PubChem | |
URL | https://pubchem.ncbi.nlm.nih.gov | |
Description | Data deposited in or computed by PubChem | |
CAS No. |
189810-94-4 | |
Record name | 5-Iodo-6-methylpyrimidine-2,4-diamine | |
Source | EPA DSSTox | |
URL | https://comptox.epa.gov/dashboard/DTXSID50578906 | |
Description | DSSTox provides a high quality public chemistry resource for supporting improved predictive toxicology. | |
Synthesis routes and methods I
Procedure details
Synthesis routes and methods II
Procedure details
Synthesis routes and methods III
Procedure details
Retrosynthesis Analysis
AI-Powered Synthesis Planning: Our tool employs the Template_relevance Pistachio, Template_relevance Bkms_metabolic, Template_relevance Pistachio_ringbreaker, Template_relevance Reaxys, Template_relevance Reaxys_biocatalysis model, leveraging a vast database of chemical reactions to predict feasible synthetic routes.
One-Step Synthesis Focus: Specifically designed for one-step synthesis, it provides concise and direct routes for your target compounds, streamlining the synthesis process.
Accurate Predictions: Utilizing the extensive PISTACHIO, BKMS_METABOLIC, PISTACHIO_RINGBREAKER, REAXYS, REAXYS_BIOCATALYSIS database, our tool offers high-accuracy predictions, reflecting the latest in chemical research and data.
Strategy Settings
Precursor scoring | Relevance Heuristic |
---|---|
Min. plausibility | 0.01 |
Model | Template_relevance |
Template Set | Pistachio/Bkms_metabolic/Pistachio_ringbreaker/Reaxys/Reaxys_biocatalysis |
Top-N result to add to graph | 6 |
Feasible Synthetic Routes
Avertissement et informations sur les produits de recherche in vitro
Veuillez noter que tous les articles et informations sur les produits présentés sur BenchChem sont destinés uniquement à des fins informatives. Les produits disponibles à l'achat sur BenchChem sont spécifiquement conçus pour des études in vitro, qui sont réalisées en dehors des organismes vivants. Les études in vitro, dérivées du terme latin "in verre", impliquent des expériences réalisées dans des environnements de laboratoire contrôlés à l'aide de cellules ou de tissus. Il est important de noter que ces produits ne sont pas classés comme médicaments et n'ont pas reçu l'approbation de la FDA pour la prévention, le traitement ou la guérison de toute condition médicale, affection ou maladie. Nous devons souligner que toute forme d'introduction corporelle de ces produits chez les humains ou les animaux est strictement interdite par la loi. Il est essentiel de respecter ces directives pour assurer la conformité aux normes légales et éthiques en matière de recherche et d'expérimentation.