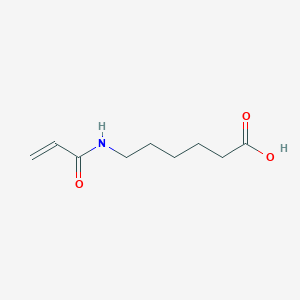
6-Acrylamidohexanoic Acid
Vue d'ensemble
Description
6-Acrylamidohexanoic Acid, also known by its CAS number 20766-85-2, is a chemical compound with the molecular formula C9H15NO3 .
Synthesis Analysis
The synthesis of this compound involves a dehydrative condensation reaction of N-hydroxysulfosuccinimide sodium salt with 6-aminohexanoic acid . The molecular weight and polydispersity indexes of the resulting polymers are well controlled by the RAFT polymerization mechanism .
Molecular Structure Analysis
The molecular structure of this compound is represented by the formula C9H15NO3 .
Chemical Reactions Analysis
This compound is used in the synthesis of polymer backbones with pendant N-hydroxysulfosuccinimide esters for post-polymerization modification in water . It is also used in the preparation of polyacrylamide gels .
Physical and Chemical Properties Analysis
This compound is a solid substance . It is soluble in methanol .
Applications De Recherche Scientifique
pH-Responsive Polymers
One notable application of AaH is in the synthesis of pH-responsive polymers. Yusa et al. (2003) synthesized well-defined poly(sodium 2-(acrylamido)-2-methylpropanesulfonate-block-sodium 6-acrylamidohexanoate) (pNaAMPS-AaH) via reversible addition−fragmentation chain transfer (RAFT) radical polymerization. These polymers exhibit pH-induced association and dissociation behavior, making them suitable for drug delivery systems where a pH-responsive mechanism is desired for targeted release (Yusa, Shimada, Mitsukami, Yamamoto, & Morishima, 2003).
Hydrogels with Self-Healing Properties
AaH is also integral in developing high-strength hydrogels with self-healing properties in both alkali and acid solutions. Wang et al. (2021) utilized AaH and [2-(methacryloyloxy)ethyl]trimethylammonium chloride solution (DMC) monomers to prepare pH-responsive hydrogels with improved mechanical properties and self-healing ability. These hydrogels have potential applications in soft robotics and as sensors for monitoring body movements (Wang, Guo, Guan, Fu, Wang, & Guo, 2021).
Environmental and Industrial Applications
In environmental and industrial contexts, AaH-based polymers are used for water treatment and as flocculants. Taeymans et al. (2004) highlighted the role of polyacrylamide, derived from acrylamide monomers like AaH, in water and wastewater treatment processes, pulp and paper processing, and mining. The study underscores the importance of understanding the chemistry and control mechanisms of acrylamide to mitigate potential health risks (Taeymans, Wood, Ashby, Blank, Studer, Stadler, Gondé, Eijck, Lalljie, Lingnert, Lindblom, Matissek, Müller, Tallmadge, O'Brien, Thompson, & Silvani, 2004).
Advanced Material Science
AaH is pivotal in creating advanced materials, such as pH-responsive 'liquid marbles' using poly(6-(acrylamido)hexanoic acid)-grafted silica particles. Inoue et al. (2011) demonstrated how these liquid marbles could burst upon exposure to alkaline conditions, suggesting potential uses in controlled delivery systems and as microreactors in chemical processes (Inoue, Fujii, Nakamura, Iwasaki, & Yusa, 2011).
Mécanisme D'action
Target of Action
It is known to act as a catalyst in compound synthesis .
Mode of Action
6-Acrylamidohexanoic Acid functions as a nucleophile, capable of donating electrons to other molecules, and also as an electrophile, capable of accepting electrons from other molecules . This dual functionality allows it to interact with a wide range of molecules, facilitating various chemical reactions.
Biochemical Pathways
It is used in the synthesis of polymers , indicating that it may play a role in polymerization reactions. In a study, it was used as a co-factor in the formation of hydrogels, suggesting its involvement in cross-linking reactions .
Pharmacokinetics
Its physical properties such as its solid form and stability at room temperature suggest that it may have good bioavailability.
Result of Action
Its role as a catalyst in compound synthesis suggests that it may facilitate the formation of complex molecules from simpler ones .
Action Environment
The action, efficacy, and stability of this compound can be influenced by various environmental factors. For instance, its stability at room temperature suggests that it can remain active under normal environmental conditions . .
Safety and Hazards
Analyse Biochimique
Biochemical Properties
6-Acrylamidohexanoic Acid plays a significant role in biochemical reactions, particularly in the synthesis of peptides and proteins. It interacts with enzymes such as N-hydroxysulfosuccinimide sodium salt, facilitating the formation of polymer backbones with reactive pendant groups . These interactions are crucial for the post-polymerization modification of polymers in aqueous solutions. The compound’s terminal carboxyl groups enable it to bind to acrylamide chains without crosslinking them, making it a valuable co-factor in various biochemical processes .
Cellular Effects
This compound influences various cellular processes, including cell signaling pathways, gene expression, and cellular metabolism. It has been shown to modulate stem cell function by affecting the mechanical properties of the cellular microenvironment . This modulation occurs through stiffness-sensitive ERK signaling pathways, which are critical for maintaining stem cell self-renewal and differentiation. Additionally, this compound’s ability to bind to acrylamide chains impacts cell adhesion and extracellular matrix interactions, further influencing cellular behavior .
Molecular Mechanism
At the molecular level, this compound exerts its effects through binding interactions with biomolecules and enzyme inhibition or activation. The compound’s interaction with N-hydroxysulfosuccinimide sodium salt leads to the formation of sulfoNHS esters, which are essential for the synthesis of glycopolymers . These glycopolymers exhibit strong interactions with lectins, such as peanut agglutinin, due to the multivalent nature of the saccharide moieties. This interaction highlights the compound’s role in modifying polymer backbones and enhancing their functionality in aqueous environments .
Temporal Effects in Laboratory Settings
In laboratory settings, the effects of this compound can change over time due to its stability and degradation properties. The compound is known to be light-sensitive, which can impact its stability and effectiveness in long-term experiments . Studies have shown that this compound remains stable under controlled conditions, but its degradation can lead to reduced efficacy in synthesizing peptides and proteins over extended periods . Understanding these temporal effects is crucial for optimizing experimental protocols and ensuring consistent results.
Dosage Effects in Animal Models
The effects of this compound vary with different dosages in animal models. At lower doses, the compound has been shown to facilitate the synthesis of peptides and proteins without causing significant adverse effects . At higher doses, it can lead to toxicity and adverse reactions, including skin and eye irritation . These dosage-dependent effects highlight the importance of careful dosage optimization in preclinical studies to balance efficacy and safety.
Metabolic Pathways
This compound is involved in various metabolic pathways, interacting with enzymes and cofactors essential for its function. The compound undergoes metabolic transformations that enable its incorporation into polymer backbones and subsequent modification . These metabolic pathways are crucial for the compound’s role in synthesizing functional polymers and enhancing their properties in biochemical applications .
Transport and Distribution
Within cells and tissues, this compound is transported and distributed through interactions with transporters and binding proteins. The compound’s solubility in methanol facilitates its uptake and distribution in aqueous environments . Additionally, its binding to acrylamide chains influences its localization and accumulation within specific cellular compartments . Understanding these transport and distribution mechanisms is essential for optimizing the compound’s use in biochemical research.
Subcellular Localization
This compound’s subcellular localization is influenced by targeting signals and post-translational modifications that direct it to specific compartments or organelles. The compound’s ability to bind to acrylamide chains and form reactive pendant groups enables its localization within the cytoplasm and extracellular matrix . These localization patterns are critical for its role in modulating cellular processes and enhancing the functionality of synthesized polymers.
Propriétés
IUPAC Name |
6-(prop-2-enoylamino)hexanoic acid | |
---|---|---|
Source | PubChem | |
URL | https://pubchem.ncbi.nlm.nih.gov | |
Description | Data deposited in or computed by PubChem | |
InChI |
InChI=1S/C9H15NO3/c1-2-8(11)10-7-5-3-4-6-9(12)13/h2H,1,3-7H2,(H,10,11)(H,12,13) | |
Source | PubChem | |
URL | https://pubchem.ncbi.nlm.nih.gov | |
Description | Data deposited in or computed by PubChem | |
InChI Key |
SAQWCPXBLNGTCC-UHFFFAOYSA-N | |
Source | PubChem | |
URL | https://pubchem.ncbi.nlm.nih.gov | |
Description | Data deposited in or computed by PubChem | |
Canonical SMILES |
C=CC(=O)NCCCCCC(=O)O | |
Source | PubChem | |
URL | https://pubchem.ncbi.nlm.nih.gov | |
Description | Data deposited in or computed by PubChem | |
Molecular Formula |
C9H15NO3 | |
Source | PubChem | |
URL | https://pubchem.ncbi.nlm.nih.gov | |
Description | Data deposited in or computed by PubChem | |
DSSTOX Substance ID |
DTXSID60314796 | |
Record name | 6-Acrylamidohexanoic Acid | |
Source | EPA DSSTox | |
URL | https://comptox.epa.gov/dashboard/DTXSID60314796 | |
Description | DSSTox provides a high quality public chemistry resource for supporting improved predictive toxicology. | |
Molecular Weight |
185.22 g/mol | |
Source | PubChem | |
URL | https://pubchem.ncbi.nlm.nih.gov | |
Description | Data deposited in or computed by PubChem | |
CAS No. |
20766-85-2 | |
Record name | 20766-85-2 | |
Source | DTP/NCI | |
URL | https://dtp.cancer.gov/dtpstandard/servlet/dwindex?searchtype=NSC&outputformat=html&searchlist=288649 | |
Description | The NCI Development Therapeutics Program (DTP) provides services and resources to the academic and private-sector research communities worldwide to facilitate the discovery and development of new cancer therapeutic agents. | |
Explanation | Unless otherwise indicated, all text within NCI products is free of copyright and may be reused without our permission. Credit the National Cancer Institute as the source. | |
Record name | 6-Acrylamidohexanoic Acid | |
Source | EPA DSSTox | |
URL | https://comptox.epa.gov/dashboard/DTXSID60314796 | |
Description | DSSTox provides a high quality public chemistry resource for supporting improved predictive toxicology. | |
Record name | 6-Acrylamidohexanoic Acid | |
Source | European Chemicals Agency (ECHA) | |
URL | https://echa.europa.eu/information-on-chemicals | |
Description | The European Chemicals Agency (ECHA) is an agency of the European Union which is the driving force among regulatory authorities in implementing the EU's groundbreaking chemicals legislation for the benefit of human health and the environment as well as for innovation and competitiveness. | |
Explanation | Use of the information, documents and data from the ECHA website is subject to the terms and conditions of this Legal Notice, and subject to other binding limitations provided for under applicable law, the information, documents and data made available on the ECHA website may be reproduced, distributed and/or used, totally or in part, for non-commercial purposes provided that ECHA is acknowledged as the source: "Source: European Chemicals Agency, http://echa.europa.eu/". Such acknowledgement must be included in each copy of the material. ECHA permits and encourages organisations and individuals to create links to the ECHA website under the following cumulative conditions: Links can only be made to webpages that provide a link to the Legal Notice page. | |
Synthesis routes and methods I
Procedure details
Synthesis routes and methods II
Procedure details
Synthesis routes and methods III
Procedure details
Synthesis routes and methods IV
Procedure details
Retrosynthesis Analysis
AI-Powered Synthesis Planning: Our tool employs the Template_relevance Pistachio, Template_relevance Bkms_metabolic, Template_relevance Pistachio_ringbreaker, Template_relevance Reaxys, Template_relevance Reaxys_biocatalysis model, leveraging a vast database of chemical reactions to predict feasible synthetic routes.
One-Step Synthesis Focus: Specifically designed for one-step synthesis, it provides concise and direct routes for your target compounds, streamlining the synthesis process.
Accurate Predictions: Utilizing the extensive PISTACHIO, BKMS_METABOLIC, PISTACHIO_RINGBREAKER, REAXYS, REAXYS_BIOCATALYSIS database, our tool offers high-accuracy predictions, reflecting the latest in chemical research and data.
Strategy Settings
Precursor scoring | Relevance Heuristic |
---|---|
Min. plausibility | 0.01 |
Model | Template_relevance |
Template Set | Pistachio/Bkms_metabolic/Pistachio_ringbreaker/Reaxys/Reaxys_biocatalysis |
Top-N result to add to graph | 6 |
Feasible Synthetic Routes
Q1: How does the incorporation of 6-acrylamidohexanoic acid (AACA) influence the properties of polyvinyl alcohol (PVA) hydrogels?
A1: Incorporating AACA into PVA hydrogels, along with a borax cross-linker, significantly enhances their mechanical strength compared to PVA hydrogels cross-linked solely with borax [, ]. This improvement stems from the introduction of multiple physical interactions within the hydrogel network. Specifically, AACA contributes to electrostatic interactions and hydrogen bonds, supplementing the existing network structure. These additional interactions contribute to the enhanced strength observed in these modified hydrogels.
Q2: Can you explain the self-healing properties observed in PVA/AACA hydrogels and their relation to pH?
A2: PVA/AACA hydrogels exhibit the intriguing property of self-healing in both acidic and alkaline conditions []. This behavior is attributed to the dynamic nature of the non-covalent interactions, such as hydrogen bonds and electrostatic interactions, present within the hydrogel structure. In acidic conditions, the carboxylic acid groups of AACA become protonated, weakening the electrostatic interactions but potentially strengthening hydrogen bonding interactions. Conversely, in alkaline environments, deprotonation of the carboxylic acid groups strengthens electrostatic interactions. This dynamic interplay of interactions allows the hydrogel network to readily reform after disruption, leading to self-healing capabilities in both acidic and alkaline environments.
Avertissement et informations sur les produits de recherche in vitro
Veuillez noter que tous les articles et informations sur les produits présentés sur BenchChem sont destinés uniquement à des fins informatives. Les produits disponibles à l'achat sur BenchChem sont spécifiquement conçus pour des études in vitro, qui sont réalisées en dehors des organismes vivants. Les études in vitro, dérivées du terme latin "in verre", impliquent des expériences réalisées dans des environnements de laboratoire contrôlés à l'aide de cellules ou de tissus. Il est important de noter que ces produits ne sont pas classés comme médicaments et n'ont pas reçu l'approbation de la FDA pour la prévention, le traitement ou la guérison de toute condition médicale, affection ou maladie. Nous devons souligner que toute forme d'introduction corporelle de ces produits chez les humains ou les animaux est strictement interdite par la loi. Il est essentiel de respecter ces directives pour assurer la conformité aux normes légales et éthiques en matière de recherche et d'expérimentation.