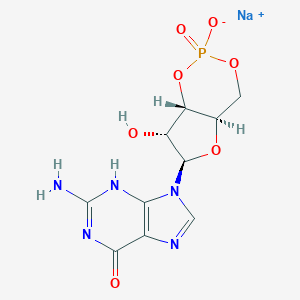
guanosine monophosphate cyclique
Vue d'ensemble
Description
Cyclic guanosine monophosphate is a cyclic nucleotide derived from guanosine triphosphate. It acts as a second messenger in various biological processes, including the regulation of ion channels, glycogenolysis, and cellular apoptosis. Cyclic guanosine monophosphate is produced by the enzyme guanylate cyclase and plays a crucial role in vascular smooth muscle relaxation, among other functions .
Applications De Recherche Scientifique
Cyclic guanosine monophosphate has a wide range of scientific research applications:
Chemistry: Used as a model compound to study nucleotide interactions and signaling pathways.
Industry: Used in the development of pharmaceuticals and as a research tool in various biochemical assays.
Mécanisme D'action
Cyclic guanosine monophosphate (cGMP), also known as Sodium guanosine cyclic monophosphate, Guanosine 3’,5’-cyclic monophosphate sodium salt, Guanosine Monophosphate Monosodium Salt, Monosodium-GMP, or Cyclic gmp, is a crucial second messenger in various physiological processes .
Target of Action
The primary targets of cGMP are the cGMP-dependent protein kinases , cyclic nucleotide-gated ion channels , and cGMP-regulated phosphodiesterases . These targets play a significant role in signal transduction, controlling a variety of physiological and pathophysiological processes in mammalian and non-mammalian tissues .
Mode of Action
cGMP acts as a second messenger, much like cyclic AMP . Its most likely mechanism of action is the activation of intracellular protein kinases in response to the binding of membrane-impermeable peptide hormones to the external cell surface . Through protein kinases activation, cGMP can relax smooth muscle .
Biochemical Pathways
cGMP is synthesized by guanylate cyclase (GC) , which converts GTP to cGMP . Peptide hormones such as the atrial natriuretic factor activate membrane-bound GC, while soluble GC (sGC) is typically activated by nitric oxide to stimulate cGMP synthesis . cGMP is a common regulator of ion channel conductance, glycogenolysis, and cellular apoptosis . It also relaxes smooth muscle tissues .
Pharmacokinetics
The intracellular cGMP levels are determined by the balance of the synthesis of this cyclic nucleotide by sGC and pGC and its hydrolysis mainly by the type 5 phosphodiesterase .
Result of Action
In blood vessels, relaxation of vascular smooth muscles leads to vasodilation and increased blood flow . At presynaptic terminals in the striatum, cGMP controls the efficacy of neurotransmitter release . In the photoreceptors of the mammalian eye, the presence of light activates phosphodiesterase, which degrades cGMP. The sodium ion channels in photoreceptors are cGMP-gated, so degradation of cGMP causes sodium channels to close, which leads to the hyperpolarization of the photoreceptor’s plasma membrane and ultimately to visual information being sent to the brain .
Action Environment
The action of cGMP can be influenced by various environmental factors. For instance, the presence of light in the environment can activate phosphodiesterase in the photoreceptors of the mammalian eye, leading to the degradation of cGMP . Furthermore, the efficacy of neurotransmitter release at presynaptic terminals in the striatum, controlled by cGMP, can also be influenced by environmental factors .
Analyse Biochimique
Biochemical Properties
Cyclic Guanosine Monophosphate Monosodium Salt plays a crucial role in various biochemical reactions. It interacts with several enzymes, proteins, and other biomolecules. The production of Cyclic Guanosine Monophosphate Monosodium Salt is triggered by the stimulation of either soluble guanylyl cyclase (sGC) or particulate guanylyl cyclase (pGC), both of which exist in different isoforms . It targets a variety of downstream effector molecules, eliciting a broad variety of cellular effects .
Cellular Effects
Cyclic Guanosine Monophosphate Monosodium Salt has significant effects on various types of cells and cellular processes. It influences cell function by impacting cell signaling pathways, gene expression, and cellular metabolism . For instance, it can cause smooth muscles and blood vessels to relax, decrease platelet aggregation, alter neuron function, etc . These effects can decrease blood pressure, increase blood flow to tissues, alter memory and behavior, decrease blood clotting, etc .
Molecular Mechanism
The mechanism of action of Cyclic Guanosine Monophosphate Monosodium Salt involves its effects at the molecular level. It exerts its effects through binding interactions with biomolecules, enzyme inhibition or activation, and changes in gene expression . Signal transduction by Cyclic Guanosine Monophosphate Monosodium Salt is controlled by the generating enzymes GCs, the degrading enzymes PDEs, and the Cyclic Guanosine Monophosphate Monosodium Salt-regulated enzymes cyclic nucleotide-gated ion channels, Cyclic Guanosine Monophosphate Monosodium Salt-dependent protein kinases, and Cyclic Guanosine Monophosphate Monosodium Salt-regulated PDEs .
Temporal Effects in Laboratory Settings
In laboratory settings, the effects of Cyclic Guanosine Monophosphate Monosodium Salt change over time. This includes information on the product’s stability, degradation, and any long-term effects on cellular function observed in in vitro or in vivo studies .
Dosage Effects in Animal Models
The effects of Cyclic Guanosine Monophosphate Monosodium Salt vary with different dosages in animal models . This includes any threshold effects observed in these studies, as well as any toxic or adverse effects at high doses .
Metabolic Pathways
Cyclic Guanosine Monophosphate Monosodium Salt is involved in several metabolic pathways. It interacts with various enzymes or cofactors . This could also include any effects on metabolic flux or metabolite levels .
Transport and Distribution
Cyclic Guanosine Monophosphate Monosodium Salt is transported and distributed within cells and tissues . This could include any transporters or binding proteins that it interacts with, as well as any effects on its localization or accumulation .
Subcellular Localization
The subcellular localization of Cyclic Guanosine Monophosphate Monosodium Salt and any effects on its activity or function could include any targeting signals or post-translational modifications that direct it to specific compartments or organelles .
Méthodes De Préparation
Synthetic Routes and Reaction Conditions
Cyclic guanosine monophosphate can be synthesized through the enzymatic conversion of guanosine triphosphate by guanylate cyclase. This enzyme catalyzes the cyclization of guanosine triphosphate to form cyclic guanosine monophosphate .
Industrial Production Methods
In industrial settings, cyclic guanosine monophosphate can be produced using engineered microbial systems. For example, Escherichia coli can be genetically modified to overexpress dinucleotide cyclases, which can then be used to produce cyclic guanosine monophosphate in large quantities. The purification process typically involves affinity chromatography and reverse-phase liquid chromatography .
Analyse Des Réactions Chimiques
Types of Reactions
Cyclic guanosine monophosphate undergoes various chemical reactions, including:
Oxidation: Cyclic guanosine monophosphate can be oxidized by reactive oxygen species, leading to the formation of oxidized derivatives.
Reduction: Although less common, cyclic guanosine monophosphate can undergo reduction under specific conditions.
Substitution: Cyclic guanosine monophosphate can participate in substitution reactions, particularly in the presence of nucleophiles.
Common Reagents and Conditions
Oxidation: Reactive oxygen species such as hydrogen peroxide can oxidize cyclic guanosine monophosphate.
Reduction: Reducing agents like sodium borohydride can be used under controlled conditions.
Substitution: Nucleophiles such as thiols can react with cyclic guanosine monophosphate in substitution reactions.
Major Products Formed
Oxidation: Oxidized derivatives of cyclic guanosine monophosphate.
Reduction: Reduced forms of cyclic guanosine monophosphate.
Substitution: Substituted cyclic guanosine monophosphate derivatives.
Comparaison Avec Des Composés Similaires
Cyclic guanosine monophosphate is often compared with other cyclic nucleotides such as cyclic adenosine monophosphate. While both act as second messengers, they have distinct roles and regulatory mechanisms:
Cyclic Adenosine Monophosphate: Primarily involved in the regulation of glycogen, sugar, and lipid metabolism.
Cyclic Inosine Monophosphate: Another cyclic nucleotide with distinct signaling roles, particularly in the regulation of vascular smooth muscle contraction.
Cyclic guanosine monophosphate’s unique ability to regulate a broad range of physiological processes, including vascular smooth muscle relaxation and cellular apoptosis, sets it apart from other cyclic nucleotides .
Propriétés
IUPAC Name |
sodium;9-[(4aR,6R,7R,7aS)-7-hydroxy-2-oxido-2-oxo-4a,6,7,7a-tetrahydro-4H-furo[3,2-d][1,3,2]dioxaphosphinin-6-yl]-2-amino-1H-purin-6-one | |
---|---|---|
Source | PubChem | |
URL | https://pubchem.ncbi.nlm.nih.gov | |
Description | Data deposited in or computed by PubChem | |
InChI |
InChI=1S/C10H12N5O7P.Na/c11-10-13-7-4(8(17)14-10)12-2-15(7)9-5(16)6-3(21-9)1-20-23(18,19)22-6;/h2-3,5-6,9,16H,1H2,(H,18,19)(H3,11,13,14,17);/q;+1/p-1/t3-,5-,6-,9-;/m1./s1 | |
Source | PubChem | |
URL | https://pubchem.ncbi.nlm.nih.gov | |
Description | Data deposited in or computed by PubChem | |
InChI Key |
KMPIYXNEROUNOG-GWTDSMLYSA-M | |
Source | PubChem | |
URL | https://pubchem.ncbi.nlm.nih.gov | |
Description | Data deposited in or computed by PubChem | |
Canonical SMILES |
C1C2C(C(C(O2)N3C=NC4=C3N=C(NC4=O)N)O)OP(=O)(O1)[O-].[Na+] | |
Source | PubChem | |
URL | https://pubchem.ncbi.nlm.nih.gov | |
Description | Data deposited in or computed by PubChem | |
Isomeric SMILES |
C1[C@@H]2[C@H]([C@H]([C@@H](O2)N3C=NC4=C3N=C(NC4=O)N)O)OP(=O)(O1)[O-].[Na+] | |
Source | PubChem | |
URL | https://pubchem.ncbi.nlm.nih.gov | |
Description | Data deposited in or computed by PubChem | |
Molecular Formula |
C10H11N5NaO7P | |
Source | PubChem | |
URL | https://pubchem.ncbi.nlm.nih.gov | |
Description | Data deposited in or computed by PubChem | |
DSSTOX Substance ID |
DTXSID10961153 | |
Record name | Guanosine monophosphate monosodium salt | |
Source | EPA DSSTox | |
URL | https://comptox.epa.gov/dashboard/DTXSID10961153 | |
Description | DSSTox provides a high quality public chemistry resource for supporting improved predictive toxicology. | |
Molecular Weight |
367.19 g/mol | |
Source | PubChem | |
URL | https://pubchem.ncbi.nlm.nih.gov | |
Description | Data deposited in or computed by PubChem | |
CAS No. |
40732-48-7 | |
Record name | Monosodium-GMP | |
Source | ChemIDplus | |
URL | https://pubchem.ncbi.nlm.nih.gov/substance/?source=chemidplus&sourceid=0040732487 | |
Description | ChemIDplus is a free, web search system that provides access to the structure and nomenclature authority files used for the identification of chemical substances cited in National Library of Medicine (NLM) databases, including the TOXNET system. | |
Record name | Guanosine monophosphate monosodium salt | |
Source | EPA DSSTox | |
URL | https://comptox.epa.gov/dashboard/DTXSID10961153 | |
Description | DSSTox provides a high quality public chemistry resource for supporting improved predictive toxicology. | |
Record name | Guanosine, cyclic 3',5'-(hydrogen phosphate), monosodium salt | |
Source | European Chemicals Agency (ECHA) | |
URL | https://echa.europa.eu/substance-information/-/substanceinfo/100.050.034 | |
Description | The European Chemicals Agency (ECHA) is an agency of the European Union which is the driving force among regulatory authorities in implementing the EU's groundbreaking chemicals legislation for the benefit of human health and the environment as well as for innovation and competitiveness. | |
Explanation | Use of the information, documents and data from the ECHA website is subject to the terms and conditions of this Legal Notice, and subject to other binding limitations provided for under applicable law, the information, documents and data made available on the ECHA website may be reproduced, distributed and/or used, totally or in part, for non-commercial purposes provided that ECHA is acknowledged as the source: "Source: European Chemicals Agency, http://echa.europa.eu/". Such acknowledgement must be included in each copy of the material. ECHA permits and encourages organisations and individuals to create links to the ECHA website under the following cumulative conditions: Links can only be made to webpages that provide a link to the Legal Notice page. | |
Record name | SODIUM GUANOSINE CYCLIC MONOPHOSPHATE | |
Source | FDA Global Substance Registration System (GSRS) | |
URL | https://gsrs.ncats.nih.gov/ginas/app/beta/substances/6WYT1TNR45 | |
Description | The FDA Global Substance Registration System (GSRS) enables the efficient and accurate exchange of information on what substances are in regulated products. Instead of relying on names, which vary across regulatory domains, countries, and regions, the GSRS knowledge base makes it possible for substances to be defined by standardized, scientific descriptions. | |
Explanation | Unless otherwise noted, the contents of the FDA website (www.fda.gov), both text and graphics, are not copyrighted. They are in the public domain and may be republished, reprinted and otherwise used freely by anyone without the need to obtain permission from FDA. Credit to the U.S. Food and Drug Administration as the source is appreciated but not required. | |
Retrosynthesis Analysis
AI-Powered Synthesis Planning: Our tool employs the Template_relevance Pistachio, Template_relevance Bkms_metabolic, Template_relevance Pistachio_ringbreaker, Template_relevance Reaxys, Template_relevance Reaxys_biocatalysis model, leveraging a vast database of chemical reactions to predict feasible synthetic routes.
One-Step Synthesis Focus: Specifically designed for one-step synthesis, it provides concise and direct routes for your target compounds, streamlining the synthesis process.
Accurate Predictions: Utilizing the extensive PISTACHIO, BKMS_METABOLIC, PISTACHIO_RINGBREAKER, REAXYS, REAXYS_BIOCATALYSIS database, our tool offers high-accuracy predictions, reflecting the latest in chemical research and data.
Strategy Settings
Precursor scoring | Relevance Heuristic |
---|---|
Min. plausibility | 0.01 |
Model | Template_relevance |
Template Set | Pistachio/Bkms_metabolic/Pistachio_ringbreaker/Reaxys/Reaxys_biocatalysis |
Top-N result to add to graph | 6 |
Feasible Synthetic Routes
ANone: Cyclic GMP exerts its effects by binding to and activating specific intracellular proteins. These targets include:
- Cyclic GMP-dependent protein kinases (PKGs): These enzymes, upon activation by cyclic GMP, phosphorylate a variety of downstream target proteins, ultimately influencing cellular processes such as smooth muscle relaxation, platelet aggregation, and neuronal signaling. [, , , , ]
- Cyclic nucleotide-gated (CNG) ion channels: Primarily found in sensory neurons (like photoreceptors) and some other cell types, these channels open upon binding cyclic GMP, leading to ion flux and changes in membrane potential. [, ]
- Cyclic GMP-regulated phosphodiesterases (PDEs): Some PDE isoforms, responsible for degrading cyclic nucleotides, are themselves regulated by cyclic GMP. This feedback loop contributes to the complex interplay between cyclic GMP and cyclic AMP signaling. [, ]
ANone: Cyclic GMP promotes relaxation of vascular smooth muscle cells through multiple mechanisms, primarily by:
- Reducing intracellular calcium levels: Cyclic GMP activates PKG, which in turn phosphorylates proteins involved in calcium extrusion and sequestration, effectively lowering cytosolic calcium and leading to relaxation. [, ]
- Modulating myosin light chain phosphorylation: PKG can directly phosphorylate myosin light chains, decreasing their sensitivity to calcium and further contributing to relaxation. [, ]
ANone: The molecular formula of cyclic GMP is C10H12N5O7P, and its molecular weight is 345.21 g/mol.
ANone: Researchers utilize a variety of model systems to dissect the complexities of cyclic GMP signaling:
- Isolated cells: Cultured cells, such as vascular smooth muscle cells, endothelial cells, and neuroblastoma cells, provide valuable tools for studying the molecular mechanisms of cyclic GMP action in a controlled environment. [, , , , ]
- Isolated tissues: Studies on isolated blood vessels, heart muscles, and tracheal smooth muscle allow for investigation of cyclic GMP's role in organ function and its interaction with other signaling pathways. [, , , , , , ]
- Animal models: Rodents, like rats and mice, are often employed to investigate the physiological and pathological roles of cyclic GMP in vivo, particularly in the context of cardiovascular diseases, neurological disorders, and retinal degeneration. [, , , ]
ANone: Researchers rely on sensitive and specific analytical techniques to measure cyclic GMP levels in various biological samples:
- Radioimmunoassay (RIA): This technique utilizes radiolabeled cyclic GMP and specific antibodies to quantify cyclic GMP concentrations in biological samples. [, , , , , ]
- Enzyme immunoassay (EIA): Similar in principle to RIA, EIA employs enzyme-linked antibodies to detect and quantify cyclic GMP. []
- High-performance liquid chromatography (HPLC): This method separates and quantifies different compounds based on their chemical properties, allowing for accurate measurement of cyclic GMP levels in complex biological matrices. []
Avertissement et informations sur les produits de recherche in vitro
Veuillez noter que tous les articles et informations sur les produits présentés sur BenchChem sont destinés uniquement à des fins informatives. Les produits disponibles à l'achat sur BenchChem sont spécifiquement conçus pour des études in vitro, qui sont réalisées en dehors des organismes vivants. Les études in vitro, dérivées du terme latin "in verre", impliquent des expériences réalisées dans des environnements de laboratoire contrôlés à l'aide de cellules ou de tissus. Il est important de noter que ces produits ne sont pas classés comme médicaments et n'ont pas reçu l'approbation de la FDA pour la prévention, le traitement ou la guérison de toute condition médicale, affection ou maladie. Nous devons souligner que toute forme d'introduction corporelle de ces produits chez les humains ou les animaux est strictement interdite par la loi. Il est essentiel de respecter ces directives pour assurer la conformité aux normes légales et éthiques en matière de recherche et d'expérimentation.