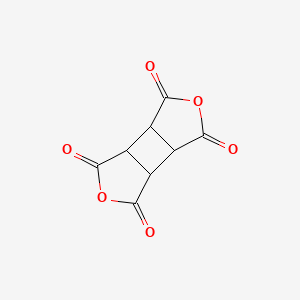
Cyclobutane-1,2,3,4-tetracarboxylic dianhydride
Vue d'ensemble
Description
Mécanisme D'action
- Its role likely involves participating in chemical reactions to form polymeric structures, such as polyimide films and liquid crystal alignment layers .
- The resulting changes involve the creation of covalent bonds, which contribute to the formation of polymer networks .
Target of Action
Mode of Action
Result of Action
Analyse Biochimique
Biochemical Properties
1,2,3,4-Cyclobutanetetracarboxylic dianhydride plays a significant role in biochemical reactions, particularly in the synthesis of biologically and pharmacologically active molecules. It interacts with various enzymes and proteins, facilitating the formation of polyimide materials. These interactions are primarily based on the compound’s ability to form stable anhydride bonds, which are crucial in the crosslinking processes of polyimides .
Cellular Effects
The effects of 1,2,3,4-Cyclobutanetetracarboxylic dianhydride on cellular processes are profound. It influences cell function by affecting cell signaling pathways, gene expression, and cellular metabolism. The compound’s interaction with cellular proteins and enzymes can lead to changes in the structural integrity of cell membranes and other cellular components, thereby impacting overall cell function .
Molecular Mechanism
At the molecular level, 1,2,3,4-Cyclobutanetetracarboxylic dianhydride exerts its effects through binding interactions with biomolecules. It can inhibit or activate enzymes, leading to alterations in gene expression. The compound’s ability to form stable anhydride bonds is central to its mechanism of action, enabling it to participate in various biochemical pathways .
Temporal Effects in Laboratory Settings
In laboratory settings, the effects of 1,2,3,4-Cyclobutanetetracarboxylic dianhydride change over time. The compound is known for its stability, but it can degrade under certain conditions, such as exposure to moisture. Long-term studies have shown that it can have lasting effects on cellular function, particularly in in vitro settings .
Dosage Effects in Animal Models
The effects of 1,2,3,4-Cyclobutanetetracarboxylic dianhydride vary with different dosages in animal models. At lower doses, the compound may facilitate beneficial biochemical reactions, while higher doses can lead to toxic or adverse effects. Threshold effects have been observed, indicating that there is a specific dosage range within which the compound is effective without being harmful .
Metabolic Pathways
1,2,3,4-Cyclobutanetetracarboxylic dianhydride is involved in several metabolic pathways. It interacts with enzymes and cofactors that are essential for the synthesis of polyimide materials. These interactions can affect metabolic flux and metabolite levels, influencing the overall metabolic state of the cell .
Transport and Distribution
Within cells and tissues, 1,2,3,4-Cyclobutanetetracarboxylic dianhydride is transported and distributed through interactions with specific transporters and binding proteins. These interactions determine the compound’s localization and accumulation, which are critical for its biochemical activity .
Subcellular Localization
The subcellular localization of 1,2,3,4-Cyclobutanetetracarboxylic dianhydride is influenced by targeting signals and post-translational modifications. These factors direct the compound to specific compartments or organelles within the cell, affecting its activity and function .
Méthodes De Préparation
Synthetic Routes and Reaction Conditions
Cyclobutane-1,2,3,4-tetracarboxylic dianhydride can be synthesized through the photochemical reaction of maleic anhydride in the presence of ethyl acetate . The reaction is carried out in a quartz glass photoreactor equipped with UV lamps (300 nm), a stirrer, and a cooler . The mixture is stirred until complete mixing is achieved .
Industrial Production Methods
In industrial settings, the production of this compound involves similar photochemical processes but on a larger scale. The use of continuous flow reactors and optimized reaction conditions ensures high yield and purity of the product .
Analyse Des Réactions Chimiques
Types of Reactions
Cyclobutane-1,2,3,4-tetracarboxylic dianhydride undergoes various chemical reactions, including:
Hydrolysis: Converts the dianhydride into the corresponding tetraacid.
Polymerization: Reacts with diamines to form polyimides.
Esterification: Forms esters when reacted with alcohols.
Common Reagents and Conditions
Hydrolysis: Water or aqueous solutions under mild conditions.
Polymerization: Diamines under high-temperature conditions.
Esterification: Alcohols in the presence of acid catalysts.
Major Products
Tetraacid: Formed from hydrolysis.
Polyimides: Formed from polymerization with diamines.
Applications De Recherche Scientifique
Cyclobutane-1,2,3,4-tetracarboxylic dianhydride has a wide range of applications in scientific research:
Chemistry: Used as a building block for synthesizing polyimides and other polymers.
Biology: Employed in the preparation of biologically active molecules.
Medicine: Utilized in drug delivery systems and as a component in medical devices.
Industry: Used in the production of high-performance organic thin-film transistors and photonic devices.
Comparaison Avec Des Composés Similaires
Similar Compounds
Maleic Anhydride: A cyclic dimer of maleic acid with similar reactivity but different structural properties.
1,2,3,4-Butanetetracarboxylic Acid: A related compound with similar functional groups but different reactivity.
Bicyclo[2.2.2]oct-7-ene-2,3,5,6-tetracarboxylic Dianhydride: Another tetracarboxylic dianhydride with distinct structural features.
Uniqueness
Cyclobutane-1,2,3,4-tetracarboxylic dianhydride is unique due to its rigid cycloaliphatic structure, which imparts high thermal stability and mechanical strength to the materials derived from it . This makes it particularly valuable in applications requiring high-performance polymers and coatings .
Propriétés
IUPAC Name |
4,9-dioxatricyclo[5.3.0.02,6]decane-3,5,8,10-tetrone | |
---|---|---|
Source | PubChem | |
URL | https://pubchem.ncbi.nlm.nih.gov | |
Description | Data deposited in or computed by PubChem | |
InChI |
InChI=1S/C8H4O6/c9-5-1-2(6(10)13-5)4-3(1)7(11)14-8(4)12/h1-4H | |
Source | PubChem | |
URL | https://pubchem.ncbi.nlm.nih.gov | |
Description | Data deposited in or computed by PubChem | |
InChI Key |
YGYCECQIOXZODZ-UHFFFAOYSA-N | |
Source | PubChem | |
URL | https://pubchem.ncbi.nlm.nih.gov | |
Description | Data deposited in or computed by PubChem | |
Canonical SMILES |
C12C(C3C1C(=O)OC3=O)C(=O)OC2=O | |
Source | PubChem | |
URL | https://pubchem.ncbi.nlm.nih.gov | |
Description | Data deposited in or computed by PubChem | |
Molecular Formula |
C8H4O6 | |
Source | PubChem | |
URL | https://pubchem.ncbi.nlm.nih.gov | |
Description | Data deposited in or computed by PubChem | |
DSSTOX Substance ID |
DTXSID00196058 | |
Record name | Tetrahydrocyclobuta(1,2-c:3,4-c')difuran-1,3,4,6-tetraone | |
Source | EPA DSSTox | |
URL | https://comptox.epa.gov/dashboard/DTXSID00196058 | |
Description | DSSTox provides a high quality public chemistry resource for supporting improved predictive toxicology. | |
Molecular Weight |
196.11 g/mol | |
Source | PubChem | |
URL | https://pubchem.ncbi.nlm.nih.gov | |
Description | Data deposited in or computed by PubChem | |
CAS No. |
4415-87-6, 4411-19-2 | |
Record name | Tetrahydrocyclobuta[1,2-c:3,4-c′]difuran-1,3,4,6-tetrone | |
Source | CAS Common Chemistry | |
URL | https://commonchemistry.cas.org/detail?cas_rn=4415-87-6 | |
Description | CAS Common Chemistry is an open community resource for accessing chemical information. Nearly 500,000 chemical substances from CAS REGISTRY cover areas of community interest, including common and frequently regulated chemicals, and those relevant to high school and undergraduate chemistry classes. This chemical information, curated by our expert scientists, is provided in alignment with our mission as a division of the American Chemical Society. | |
Explanation | The data from CAS Common Chemistry is provided under a CC-BY-NC 4.0 license, unless otherwise stated. | |
Record name | 1,2,3,4-Cyclobutanetetracarboxylic dianhydride | |
Source | ChemIDplus | |
URL | https://pubchem.ncbi.nlm.nih.gov/substance/?source=chemidplus&sourceid=0004415876 | |
Description | ChemIDplus is a free, web search system that provides access to the structure and nomenclature authority files used for the identification of chemical substances cited in National Library of Medicine (NLM) databases, including the TOXNET system. | |
Record name | NSC131455 | |
Source | DTP/NCI | |
URL | https://dtp.cancer.gov/dtpstandard/servlet/dwindex?searchtype=NSC&outputformat=html&searchlist=131455 | |
Description | The NCI Development Therapeutics Program (DTP) provides services and resources to the academic and private-sector research communities worldwide to facilitate the discovery and development of new cancer therapeutic agents. | |
Explanation | Unless otherwise indicated, all text within NCI products is free of copyright and may be reused without our permission. Credit the National Cancer Institute as the source. | |
Record name | Tetrahydrocyclobuta(1,2-c:3,4-c')difuran-1,3,4,6-tetraone | |
Source | EPA DSSTox | |
URL | https://comptox.epa.gov/dashboard/DTXSID00196058 | |
Description | DSSTox provides a high quality public chemistry resource for supporting improved predictive toxicology. | |
Record name | Tetrahydrocyclobuta[1,2-c:3,4-c']difuran-1,3,4,6-tetraone | |
Source | European Chemicals Agency (ECHA) | |
URL | https://echa.europa.eu/substance-information/-/substanceinfo/100.022.344 | |
Description | The European Chemicals Agency (ECHA) is an agency of the European Union which is the driving force among regulatory authorities in implementing the EU's groundbreaking chemicals legislation for the benefit of human health and the environment as well as for innovation and competitiveness. | |
Explanation | Use of the information, documents and data from the ECHA website is subject to the terms and conditions of this Legal Notice, and subject to other binding limitations provided for under applicable law, the information, documents and data made available on the ECHA website may be reproduced, distributed and/or used, totally or in part, for non-commercial purposes provided that ECHA is acknowledged as the source: "Source: European Chemicals Agency, http://echa.europa.eu/". Such acknowledgement must be included in each copy of the material. ECHA permits and encourages organisations and individuals to create links to the ECHA website under the following cumulative conditions: Links can only be made to webpages that provide a link to the Legal Notice page. | |
Record name | 1,2,3,4-CYCLOBUTANETETRACARBOXYLIC DIANHYDRIDE | |
Source | FDA Global Substance Registration System (GSRS) | |
URL | https://gsrs.ncats.nih.gov/ginas/app/beta/substances/P1DOQ7P28N | |
Description | The FDA Global Substance Registration System (GSRS) enables the efficient and accurate exchange of information on what substances are in regulated products. Instead of relying on names, which vary across regulatory domains, countries, and regions, the GSRS knowledge base makes it possible for substances to be defined by standardized, scientific descriptions. | |
Explanation | Unless otherwise noted, the contents of the FDA website (www.fda.gov), both text and graphics, are not copyrighted. They are in the public domain and may be republished, reprinted and otherwise used freely by anyone without the need to obtain permission from FDA. Credit to the U.S. Food and Drug Administration as the source is appreciated but not required. | |
Synthesis routes and methods I
Procedure details
Synthesis routes and methods II
Procedure details
Retrosynthesis Analysis
AI-Powered Synthesis Planning: Our tool employs the Template_relevance Pistachio, Template_relevance Bkms_metabolic, Template_relevance Pistachio_ringbreaker, Template_relevance Reaxys, Template_relevance Reaxys_biocatalysis model, leveraging a vast database of chemical reactions to predict feasible synthetic routes.
One-Step Synthesis Focus: Specifically designed for one-step synthesis, it provides concise and direct routes for your target compounds, streamlining the synthesis process.
Accurate Predictions: Utilizing the extensive PISTACHIO, BKMS_METABOLIC, PISTACHIO_RINGBREAKER, REAXYS, REAXYS_BIOCATALYSIS database, our tool offers high-accuracy predictions, reflecting the latest in chemical research and data.
Strategy Settings
Precursor scoring | Relevance Heuristic |
---|---|
Min. plausibility | 0.01 |
Model | Template_relevance |
Template Set | Pistachio/Bkms_metabolic/Pistachio_ringbreaker/Reaxys/Reaxys_biocatalysis |
Top-N result to add to graph | 6 |
Feasible Synthetic Routes
Q1: What is the molecular formula and weight of CBDA?
A1: The molecular formula of CBDA is C8H6O6, and its molecular weight is 202.12 g/mol.
Q2: Does CBDA exhibit any unique structural features compared to other dianhydrides?
A2: Yes, CBDA possesses a unique cyclobutane ring structure, contributing to the low coefficient of thermal expansion (CTE) observed in its derived polymers [, , , ].
Q3: What spectroscopic data is available to characterize CBDA?
A3: CBDA can be characterized using various spectroscopic techniques, primarily Fourier Transform Infrared Spectroscopy (FTIR) and Nuclear Magnetic Resonance (NMR) spectroscopy. FTIR reveals characteristic peaks for the anhydride groups and the cyclobutane ring, while NMR provides detailed information about the carbon and hydrogen atoms in the molecule [, , , ].
Q4: How does CBDA contribute to the solubility of its derived polymers?
A4: While CBDA itself may have limited solubility, its incorporation into polymer backbones, particularly polyimides, can significantly influence solubility. For instance, CBDA-based PIs incorporating fluorinated aromatic diamines have demonstrated enhanced solubility in various organic solvents [, ].
Q5: How does CBDA impact the thermal stability of its derived polymers?
A5: CBDA generally contributes to good thermal stability in its derived polymers. For example, PIs synthesized from CBDA and various diamines have shown high thermal decomposition temperatures, often exceeding 400°C [, , , ].
Q6: How does the steric structure of CBDA influence the properties of derived polyimides?
A6: The rigid and compact cyclobutane ring in CBDA significantly impacts the polymer chain conformation and packing, ultimately influencing properties such as CTE, glass transition temperature (Tg), and solubility. This impact is evident when comparing CBDA-based PIs with those derived from other dianhydrides with different steric structures [, , ].
Q7: What are the advantages of using CBDA in synthesizing polyimides for optoelectronic applications?
A7: CBDA-based PIs often exhibit excellent optical transparency, particularly in the visible light region, making them suitable for optoelectronic applications. This transparency, combined with their high thermal stability and often low CTE, makes them ideal for applications requiring demanding thermal and optical properties [, , ].
Q8: Are there any challenges associated with using CBDA in polymer synthesis?
A8: Yes, polymerization with CBDA can be challenging due to potential salt formation with certain diamines, impacting molecular weight and reaction time. This challenge is particularly prominent with trans-1,4-cyclohexanediamine (t-CHDA) and can be addressed by controlled heating methods during polymerization [, ].
Q9: What types of polymers are commonly synthesized using CBDA?
A9: CBDA is widely employed in synthesizing high-performance polyimides, particularly for applications demanding low CTE, high Tg, and good optical transparency. It is also used in preparing poly(amic acid)s (PAAs), the precursors to polyimides [, , , , , , ].
Q10: Can CBDA be used in synthesizing polymers other than polyimides?
A10: Yes, CBDA has been explored in the synthesis of other polymers, including poly(ether-benzoxazole)s (PEBOs), polyimidosiloxanes (APISiOs), and poly(pyridine–imide)s [, , ].
Q11: How does CBDA influence the properties of photo-crosslinkable poly(amic acid ester)s (PAEs)?
A11: CBDA contributes to the thermal stability and photosensitivity of PAEs. For example, PAEs synthesized from CBDA, 4,4′-diaminodiphenyl ether, and a photosensitive component have shown good resolution after photolithography and high thermal stability in the final cured polyimide films [, ].
Q12: Can CBDA be used to modify existing polymers?
A12: Yes, while not as common as its use in de novo polymer synthesis, CBDA can be employed for polymer modification. For instance, it has been used to create dynamic covalent bonds in aliphatic polyester elastomers, enabling reprocessability and tunable mechanical properties [].
Q13: How does the incorporation of CBDA influence the liquid crystal (LC) alignment properties of polyimides?
A13: CBDA plays a crucial role in controlling the pretilt angle of LC molecules in LC alignment layers. This influence is attributed to the specific interactions between the CBDA-derived PI surface and the LC molecules, which can be further tuned by modifying the PI structure or adding specific additives [, , ].
Q14: Have there been any computational studies conducted on CBDA and its derived polymers?
A14: Yes, computational chemistry methods, such as density functional theory (DFT) calculations, have been employed to study the electronic structure, optical properties, and thermal stability of CBDA-based polymers [, , ].
Q15: How do structure-activity relationship (SAR) studies inform the design of CBDA-based polymers?
A15: SAR studies are crucial for understanding how modifications to the CBDA structure or the co-monomers impact the final polymer properties. This knowledge allows for the rational design of polymers with tailored characteristics, such as enhanced solubility, tailored CTE, or specific optical properties [, , , , , ].
Q16: Is there any information available on the environmental impact and degradation of CBDA and its derived polymers?
A16: While specific data on CBDA's environmental impact might be limited, research on related cycloaliphatic compounds suggests potential biodegradability []. Further research is needed to assess the environmental fate and potential ecotoxicological effects of CBDA and its derived polymers [].
Q17: Are there any strategies for recycling or managing the waste generated from CBDA-based polymers?
A17: Research into the recycling and waste management of CBDA-based polymers is ongoing. Potential strategies include exploring chemical recycling methods to depolymerize the materials and recover monomers, as well as developing biodegradable or compostable analogs [, ].
Avertissement et informations sur les produits de recherche in vitro
Veuillez noter que tous les articles et informations sur les produits présentés sur BenchChem sont destinés uniquement à des fins informatives. Les produits disponibles à l'achat sur BenchChem sont spécifiquement conçus pour des études in vitro, qui sont réalisées en dehors des organismes vivants. Les études in vitro, dérivées du terme latin "in verre", impliquent des expériences réalisées dans des environnements de laboratoire contrôlés à l'aide de cellules ou de tissus. Il est important de noter que ces produits ne sont pas classés comme médicaments et n'ont pas reçu l'approbation de la FDA pour la prévention, le traitement ou la guérison de toute condition médicale, affection ou maladie. Nous devons souligner que toute forme d'introduction corporelle de ces produits chez les humains ou les animaux est strictement interdite par la loi. Il est essentiel de respecter ces directives pour assurer la conformité aux normes légales et éthiques en matière de recherche et d'expérimentation.