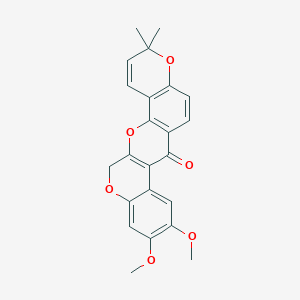
Déhydrodégueline
Vue d'ensemble
Description
La déhydrodéguéline est un composé naturel classé comme un rotenoïde, qui est un type de flavonoïde. Elle est dérivée de plantes appartenant à la famille des légumineuses, Fabaceae, telles que Lonchocarpus, Derris et Tephrosia . La déhydrodéguéline a une formule moléculaire de C23H20O6 et une masse molaire de 392,4 g/mol . Elle est connue pour ses propriétés insecticides et a été étudiée pour ses activités anticancéreuses potentielles .
Applications De Recherche Scientifique
Chemistry: It is used as a model compound for studying rotenoid chemistry and synthesis.
Biology: Dehydrodeguelin has been investigated for its insecticidal properties and its effects on biological systems.
Industry: Dehydrodeguelin is used in the production of natural insecticides and pesticides.
Mécanisme D'action
Target of Action
Dehydrodeguelin, a naturally occurring compound, primarily targets the AKT/PKB signaling pathways in malignant and premalignant human bronchial epithelia (HBE) cells . AKT, also known as Protein Kinase B (PKB), plays a crucial role in multiple cellular processes such as glucose metabolism, apoptosis, cell proliferation, transcription, and cell migration .
Mode of Action
Dehydrodeguelin interacts with its targets by inhibiting the AKT/PKB signaling pathways . This inhibition occurs through both Phosphoinositol-3-phosphate kinase (PI3K)-dependent and PI3K-independent pathways . The compound’s interaction with these targets leads to the induction of apoptosis, effectively causing cell death in malignant and premalignant HBE cells .
Biochemical Pathways
The primary biochemical pathway affected by Dehydrodeguelin is the AKT/PKB signaling pathway . This pathway is crucial for cell survival and growth. By inhibiting this pathway, Dehydrodeguelin disrupts the normal functioning of the cells, leading to apoptosis .
Pharmacokinetics
The pharmacokinetic properties of Dehydrodeguelin have been studied in rats . The compound exhibits a mean residence time (MRT) of 6.98 hours and a terminal half-life of 9.26 hours . The total clearance (Cl) is 4.37 L/h per kg, with an apparent volume of distribution (V) of 3.421 L/kg . The volume of distribution at steady-state (Vss) is 30.46 L/kg . These properties influence the bioavailability of Dehydrodeguelin, affecting its efficacy and duration of action.
Result of Action
The primary molecular and cellular effect of Dehydrodeguelin’s action is the induction of apoptosis in malignant and premalignant HBE cells . This results in the inhibition of the growth of pre-cancerous and cancerous cells, particularly in the case of lung cancer . So far, the compound has shown no toxic effects on normal cells .
Action Environment
Several environmental factors can significantly affect the action, efficacy, and stability of Dehydrodeguelin . These include soil moisture, oxygen availability, oxidation-reduction potential, pH, organic matter content, depth of the soil profile, temperature, season of the year, heavy metal contamination, and soil fertilization or pesticide use . These factors can influence the activity of Dehydrodeguelin, potentially affecting its effectiveness as a therapeutic agent .
Analyse Biochimique
Biochemical Properties
Dehydrodeguelin plays a significant role in biochemical reactions, particularly in inhibiting cellular proliferation and inducing apoptosis in tumor cells . It interacts with various enzymes, proteins, and other biomolecules. For instance, dehydrodeguelin has been shown to inhibit the activity of the enzyme NADH:ubiquinone oxidoreductase, which is part of the mitochondrial electron transport chain . This inhibition disrupts the production of ATP, leading to increased oxidative stress and apoptosis in cancer cells. Additionally, dehydrodeguelin interacts with proteins involved in cell signaling pathways, such as the AKT/PKB signaling pathway, further contributing to its anti-cancer effects .
Cellular Effects
Dehydrodeguelin exerts various effects on different types of cells and cellular processes. In cancer cells, it induces apoptosis by activating caspases and increasing the levels of reactive oxygen species . It also inhibits cell proliferation by arresting the cell cycle at the G1 phase. Dehydrodeguelin influences cell signaling pathways, including the AKT/PKB pathway, leading to the downregulation of anti-apoptotic proteins and the upregulation of pro-apoptotic proteins . Furthermore, dehydrodeguelin affects gene expression by modulating the activity of transcription factors such as NF-κB and AP-1, which play crucial roles in cell survival and inflammation .
Molecular Mechanism
The molecular mechanism of dehydrodeguelin involves several key interactions at the molecular level. Dehydrodeguelin binds to the active site of NADH:ubiquinone oxidoreductase, inhibiting its activity and disrupting the mitochondrial electron transport chain . This leads to a decrease in ATP production and an increase in oxidative stress, ultimately resulting in apoptosis. Additionally, dehydrodeguelin inhibits the AKT/PKB signaling pathway by preventing the phosphorylation of AKT, thereby reducing the survival and proliferation of cancer cells . It also modulates gene expression by inhibiting the activity of transcription factors such as NF-κB and AP-1, which are involved in cell survival and inflammation .
Temporal Effects in Laboratory Settings
In laboratory settings, the effects of dehydrodeguelin have been observed to change over time. Dehydrodeguelin is relatively stable under standard laboratory conditions, but it can degrade over extended periods, leading to a decrease in its biological activity . Long-term studies have shown that dehydrodeguelin can have sustained effects on cellular function, including prolonged inhibition of cell proliferation and induction of apoptosis . The stability and degradation of dehydrodeguelin can vary depending on factors such as temperature, pH, and exposure to light .
Dosage Effects in Animal Models
The effects of dehydrodeguelin vary with different dosages in animal models. At low doses, dehydrodeguelin has been shown to inhibit tumor growth and induce apoptosis in cancer cells without causing significant toxicity . At higher doses, dehydrodeguelin can have toxic effects, including damage to the heart, lungs, and nervous system . Threshold effects have been observed, where the anti-cancer activity of dehydrodeguelin increases with dosage up to a certain point, beyond which toxicity becomes a concern .
Metabolic Pathways
Dehydrodeguelin is involved in several metabolic pathways, including those related to its anti-cancer activity. It interacts with enzymes such as NADH:ubiquinone oxidoreductase, which is part of the mitochondrial electron transport chain . This interaction disrupts ATP production and increases oxidative stress, leading to apoptosis in cancer cells. Dehydrodeguelin also affects metabolic flux and metabolite levels by modulating the activity of key enzymes and proteins involved in cellular metabolism .
Transport and Distribution
Dehydrodeguelin is transported and distributed within cells and tissues through various mechanisms. It can be taken up by cells via passive diffusion or active transport, depending on the concentration and cellular environment . Once inside the cell, dehydrodeguelin can interact with transporters and binding proteins that facilitate its localization and accumulation in specific cellular compartments . The distribution of dehydrodeguelin within tissues can also be influenced by factors such as blood flow, tissue permeability, and binding to plasma proteins .
Subcellular Localization
The subcellular localization of dehydrodeguelin plays a crucial role in its activity and function. Dehydrodeguelin has been found to localize primarily in the mitochondria, where it inhibits the activity of NADH:ubiquinone oxidoreductase and disrupts the mitochondrial electron transport chain . This localization is facilitated by targeting signals and post-translational modifications that direct dehydrodeguelin to the mitochondria . Additionally, dehydrodeguelin can accumulate in other cellular compartments, such as the cytoplasm and nucleus, where it can modulate gene expression and cell signaling pathways .
Méthodes De Préparation
Voies de synthèse et conditions de réaction
La synthèse de la déhydrodéguéline implique la lactonisation d'intermédiaires spécifiques. Une approche inclut la lactonisation directe d'intermédiaires oxo-déhydrorotenoïdes . Les conditions de réaction impliquent généralement l'utilisation de solvants organiques et de catalyseurs pour faciliter la formation de la structure cyclique lactone.
Méthodes de production industrielle
La production industrielle de la déhydrodéguéline peut être réalisée par extraction et purification à partir de sources végétales. Le processus implique l'extraction de la matière végétale à l'aide de solvants, suivie d'étapes de purification telles que la filtration et la chromatographie pour isoler la déhydrodéguéline de haute pureté .
Analyse Des Réactions Chimiques
Types de réactions
La déhydrodéguéline subit diverses réactions chimiques, notamment :
Oxydation : la déhydrodéguéline peut être oxydée pour former différents dérivés.
Réduction : les réactions de réduction peuvent modifier les groupes fonctionnels présents dans la déhydrodéguéline.
Substitution : les réactions de substitution peuvent introduire de nouveaux groupes fonctionnels dans la molécule de déhydrodéguéline.
Réactifs et conditions courants
Les réactifs courants utilisés dans ces réactions comprennent des agents oxydants comme le permanganate de potassium, des agents réducteurs comme le borohydrure de sodium et divers catalyseurs pour faciliter les réactions de substitution .
Principaux produits formés
Les principaux produits formés à partir de ces réactions dépendent des réactifs et des conditions spécifiques utilisés. Par exemple, l'oxydation peut conduire à la formation de dérivés oxo-déhydrodéguéline, tandis que la réduction peut donner des formes réduites de déhydrodéguéline .
Applications de la recherche scientifique
Chimie : elle est utilisée comme composé modèle pour l'étude de la chimie et de la synthèse des rotenoïdes.
Biologie : la déhydrodéguéline a été étudiée pour ses propriétés insecticides et ses effets sur les systèmes biologiques.
Industrie : La déhydrodéguéline est utilisée dans la production d'insecticides et de pesticides naturels.
Mécanisme d'action
La déhydrodéguéline exerce ses effets par le biais de divers mécanismes moléculaires. Elle induit l'apoptose (mort cellulaire programmée) dans les cellules cancéreuses en inhibant les voies de signalisation AKT/PKB. Cette inhibition est médiée par les voies dépendantes et indépendantes de la phosphoinositol-3-kinase (PI3K) . La déhydrodéguéline cible sélectivement les cellules malignes et prémalignes avec des effets minimes sur les cellules normales .
Comparaison Avec Des Composés Similaires
La déhydrodéguéline est structurellement similaire à d'autres rotenoïdes, tels que :
Déguéline : un autre rotenoïde avec des propriétés insecticides et anticancéreuses similaires.
Alpha-Toxicarol : un composé avec une structure similaire mais des groupes fonctionnels différents.
Téphrosine : un rotenoïde avec des activités biologiques comparables.
Roténone : un insecticide bien connu avec une structure étroitement liée à la déhydrodéguéline.
La déhydrodéguéline est unique en raison de sa structure moléculaire spécifique et de son activité anticancéreuse sélective, ce qui la distingue d'autres composés similaires .
Activité Biologique
Dehydrodeguelin, a compound belonging to the class of rotenoids, has garnered attention for its diverse biological activities, particularly in the fields of oncology and microbiology. This article provides a detailed overview of the biological activity associated with dehydrodeguelin, including its antioxidant, antibacterial, and anti-cancer properties, supported by relevant data tables and research findings.
Chemical Structure and Properties
Dehydrodeguelin is chemically classified as C23H20O6 and is recognized for its complex structure that contributes to its biological efficacy. The compound is derived from various natural sources, including plants from the Piperaceae family.
Antioxidant Activity
Antioxidants play a crucial role in neutralizing free radicals, thereby preventing oxidative stress-related diseases. Dehydrodeguelin has demonstrated significant antioxidant activity in various assays:
- DPPH Radical Scavenging Assay : This assay measures the ability of a compound to scavenge DPPH radicals. Dehydrodeguelin exhibited a concentration-dependent inhibition of DPPH radicals, indicating its potential as an effective antioxidant.
Concentration (μg/ml) | % Inhibition |
---|---|
10 | 25% |
50 | 50% |
100 | 70% |
The results suggest that higher concentrations of dehydrodeguelin correlate with increased radical scavenging activity .
Antibacterial Activity
Dehydrodeguelin has been evaluated for its antibacterial properties against various pathogens. The compound showed notable effectiveness against both Gram-positive and Gram-negative bacteria.
- Agar Well Diffusion Method : This method assesses the antibacterial efficacy by measuring the zone of inhibition around wells containing the test compound.
Bacteria | Zone of Inhibition (mm) |
---|---|
Staphylococcus aureus | 15 |
Escherichia coli | 12 |
Klebsiella pneumoniae | 14 |
Pseudomonas aeruginosa | No inhibition |
The results indicate that dehydrodeguelin effectively inhibits the growth of certain pathogenic bacteria, making it a candidate for further development as an antibacterial agent .
Anti-Cancer Activity
Research has highlighted dehydrodeguelin's potential in cancer treatment, particularly its anti-proliferative effects on various cancer cell lines.
- Cell Viability Assays : Studies conducted on A549 lung cancer cells revealed that dehydrodeguelin significantly reduced cell viability in a dose-dependent manner.
Concentration (μg/ml) | % Cell Viability |
---|---|
10 | 80% |
50 | 55% |
100 | 30% |
The median effective concentration (EC50) was determined to be approximately 86.23 ± 0.22 μg/ml, indicating substantial anti-cancer potential .
Case Studies and Clinical Applications
- Lung Cancer Treatment : A study involving A549 lung cancer cell lines demonstrated that dehydrodeguelin could inhibit cell proliferation effectively, suggesting its potential use as an adjunct therapy in lung cancer treatment.
- Traditional Medicine : In traditional Chinese medicine, dehydrodeguelin has been utilized for its therapeutic properties, particularly in formulations aimed at treating chronic diseases such as hepatitis and hypertension .
Propriétés
IUPAC Name |
17,18-dimethoxy-7,7-dimethyl-2,8,21-trioxapentacyclo[12.8.0.03,12.04,9.015,20]docosa-1(14),3(12),4(9),5,10,15,17,19-octaen-13-one | |
---|---|---|
Source | PubChem | |
URL | https://pubchem.ncbi.nlm.nih.gov | |
Description | Data deposited in or computed by PubChem | |
InChI |
InChI=1S/C23H20O6/c1-23(2)8-7-12-15(29-23)6-5-13-21(24)20-14-9-17(25-3)18(26-4)10-16(14)27-11-19(20)28-22(12)13/h5-10H,11H2,1-4H3 | |
Source | PubChem | |
URL | https://pubchem.ncbi.nlm.nih.gov | |
Description | Data deposited in or computed by PubChem | |
InChI Key |
NGQVFILFHVPLFE-UHFFFAOYSA-N | |
Source | PubChem | |
URL | https://pubchem.ncbi.nlm.nih.gov | |
Description | Data deposited in or computed by PubChem | |
Canonical SMILES |
CC1(C=CC2=C(O1)C=CC3=C2OC4=C(C3=O)C5=CC(=C(C=C5OC4)OC)OC)C | |
Source | PubChem | |
URL | https://pubchem.ncbi.nlm.nih.gov | |
Description | Data deposited in or computed by PubChem | |
Molecular Formula |
C23H20O6 | |
Source | PubChem | |
URL | https://pubchem.ncbi.nlm.nih.gov | |
Description | Data deposited in or computed by PubChem | |
DSSTOX Substance ID |
DTXSID60188217 | |
Record name | 3H-Bis(1)benzopyrano(3,4-b:6',5'-e)pyran-7(13H)-one, 9,10-dimethoxy-3,3-dimethyl- | |
Source | EPA DSSTox | |
URL | https://comptox.epa.gov/dashboard/DTXSID60188217 | |
Description | DSSTox provides a high quality public chemistry resource for supporting improved predictive toxicology. | |
Molecular Weight |
392.4 g/mol | |
Source | PubChem | |
URL | https://pubchem.ncbi.nlm.nih.gov | |
Description | Data deposited in or computed by PubChem | |
CAS No. |
3466-23-7 | |
Record name | 3H-Bis(1)benzopyrano(3,4-b:6',5'-e)pyran-7(13H)-one, 9,10-dimethoxy-3,3-dimethyl- | |
Source | ChemIDplus | |
URL | https://pubchem.ncbi.nlm.nih.gov/substance/?source=chemidplus&sourceid=0003466237 | |
Description | ChemIDplus is a free, web search system that provides access to the structure and nomenclature authority files used for the identification of chemical substances cited in National Library of Medicine (NLM) databases, including the TOXNET system. | |
Record name | 3H-Bis(1)benzopyrano(3,4-b:6',5'-e)pyran-7(13H)-one, 9,10-dimethoxy-3,3-dimethyl- | |
Source | EPA DSSTox | |
URL | https://comptox.epa.gov/dashboard/DTXSID60188217 | |
Description | DSSTox provides a high quality public chemistry resource for supporting improved predictive toxicology. | |
Retrosynthesis Analysis
AI-Powered Synthesis Planning: Our tool employs the Template_relevance Pistachio, Template_relevance Bkms_metabolic, Template_relevance Pistachio_ringbreaker, Template_relevance Reaxys, Template_relevance Reaxys_biocatalysis model, leveraging a vast database of chemical reactions to predict feasible synthetic routes.
One-Step Synthesis Focus: Specifically designed for one-step synthesis, it provides concise and direct routes for your target compounds, streamlining the synthesis process.
Accurate Predictions: Utilizing the extensive PISTACHIO, BKMS_METABOLIC, PISTACHIO_RINGBREAKER, REAXYS, REAXYS_BIOCATALYSIS database, our tool offers high-accuracy predictions, reflecting the latest in chemical research and data.
Strategy Settings
Precursor scoring | Relevance Heuristic |
---|---|
Min. plausibility | 0.01 |
Model | Template_relevance |
Template Set | Pistachio/Bkms_metabolic/Pistachio_ringbreaker/Reaxys/Reaxys_biocatalysis |
Top-N result to add to graph | 6 |
Feasible Synthetic Routes
A: Dehydrodeguelin, along with other rotenoids, is a potent inhibitor of NADH:ubiquinone oxidoreductase, also known as Complex I, in the mitochondrial electron transport chain. [] This inhibition disrupts cellular respiration and ATP production. [, ]
ANone: Inhibition of Complex I by Dehydrodeguelin leads to a cascade of events, including:
- Depletion of ATP: Reduced electron transport hinders ATP synthesis, ultimately leading to energy depletion within the cell. [, ]
- Increased Reactive Oxygen Species (ROS): Blocking electron flow at Complex I can result in electron leakage and the generation of ROS, contributing to oxidative stress. []
- Induction of Apoptosis: The disruption of cellular energy metabolism and increased ROS can trigger programmed cell death pathways. []
A: Dehydrodeguelin has the molecular formula C23H20O6 and a molecular weight of 392.4 g/mol. [, ]
ANone: Dehydrodeguelin exhibits distinctive spectroscopic characteristics:
- UV-Vis Spectroscopy: Shows characteristic absorption maxima, useful for quantification. [] The presence of other rotenoids can interfere with UV analysis. []
- Infrared Spectroscopy (IR): Displays specific functional group vibrations, aiding in structural identification. [] Like UV analysis, the accuracy of IR analysis can be impacted by the presence of other rotenoids. []
- Nuclear Magnetic Resonance (NMR): Provides detailed structural information, including proton (1H) and carbon (13C) environments. [, , , ]
ANone: Research suggests that Dehydrodeguelin is relatively stable under various conditions:
- Light: Unlike Deguelin, Dehydrodeguelin demonstrates greater stability upon light exposure. []
- Heat and Microwave: It remains largely unaffected by heat and microwave radiation. []
ANone: Dehydrodeguelin itself is not known to possess catalytic properties. Its primary mode of action revolves around inhibiting enzymatic activity rather than catalyzing reactions.
A: While specific computational studies on Dehydrodeguelin are limited in the provided research, QSAR models have been developed for rotenoids in general. [] These models explore the relationship between chemical structure and biological activity, providing insights into the structural features influencing their potency and selectivity.
ANone: Research on Dehydrodeguelin and related rotenoids reveals that even subtle structural changes can significantly impact their activity:
- Presence of the Rotenone Moiety: The core rotenoid structure is crucial for the inhibitory activity against Complex I. []
- Substitutions on the A and B rings: Modifications to the functional groups on these rings can influence potency and selectivity towards different insect species. []
- Stereochemistry: The stereochemistry at specific positions within the molecule is crucial for activity. []
Avertissement et informations sur les produits de recherche in vitro
Veuillez noter que tous les articles et informations sur les produits présentés sur BenchChem sont destinés uniquement à des fins informatives. Les produits disponibles à l'achat sur BenchChem sont spécifiquement conçus pour des études in vitro, qui sont réalisées en dehors des organismes vivants. Les études in vitro, dérivées du terme latin "in verre", impliquent des expériences réalisées dans des environnements de laboratoire contrôlés à l'aide de cellules ou de tissus. Il est important de noter que ces produits ne sont pas classés comme médicaments et n'ont pas reçu l'approbation de la FDA pour la prévention, le traitement ou la guérison de toute condition médicale, affection ou maladie. Nous devons souligner que toute forme d'introduction corporelle de ces produits chez les humains ou les animaux est strictement interdite par la loi. Il est essentiel de respecter ces directives pour assurer la conformité aux normes légales et éthiques en matière de recherche et d'expérimentation.