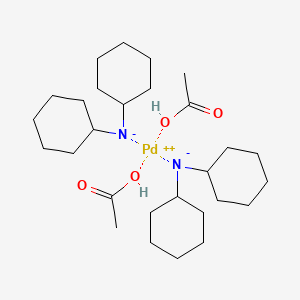
Acétate de trans-bis(dicyclohexylamine)palladium(II)
Vue d'ensemble
Description
Trans-Bis(dicyclohexylamine)palladium(II) acetate, also known as trans-BDCP, is a palladium-containing organometallic compound that has been used in a variety of applications, including catalysis, biochemistry, and drug development. It is a versatile compound that has been studied extensively in the laboratory, and its unique properties make it a valuable tool for researchers and scientists.
Applications De Recherche Scientifique
Voici une analyse complète des applications de recherche scientifique de l'acétate de trans-bis(dicyclohexylamine)palladium(II) :
Couplage de Negishi
Il catalyse les réactions de couplage de Negishi qui sont utilisées pour former des liaisons carbone-carbone, en particulier dans la synthèse organique complexe.
Chaque application utilise les propriétés catalytiques de l'acétate de trans-bis(dicyclohexylamine)palladium(II) pour faciliter différents types de réactions chimiques qui sont fondamentales dans divers domaines de la recherche scientifique et de l'industrie .
Mécanisme D'action
Target of Action
The primary target of trans-Bis(dicyclohexylamine)palladium(II) acetate is the formation of carbon-carbon and carbon-nitrogen bonds . This compound is a catalyst that facilitates these bond formations in various types of coupling reactions .
Mode of Action
trans-Bis(dicyclohexylamine)palladium(II) acetate: interacts with its targets by accelerating the rate of bond formation. It does this by providing a lower energy pathway for the reaction, thus enabling the reaction to proceed more quickly and efficiently .
Biochemical Pathways
The trans-Bis(dicyclohexylamine)palladium(II) acetate affects the biochemical pathways involved in the synthesis of complex organic molecules. By facilitating the formation of carbon-carbon and carbon-nitrogen bonds, it plays a crucial role in the construction of these molecules . The downstream effects include the synthesis of a wide range of organic compounds, including pharmaceuticals and polymers .
Pharmacokinetics
The ADME properties of trans-Bis(dicyclohexylamine)palladium(II) acetate Its effectiveness can be influenced by its solubility and stability under the reaction conditions .
Result of Action
The molecular and cellular effects of trans-Bis(dicyclohexylamine)palladium(II) acetate ’s action are seen in the products of the reactions it catalyzes. These can include a wide range of organic compounds, depending on the specific reactants used in the coupling reaction .
Action Environment
The action, efficacy, and stability of trans-Bis(dicyclohexylamine)palladium(II) acetate can be influenced by various environmental factors. These include the temperature and pH of the reaction environment, the presence of other substances that can coordinate with the palladium center, and the solvent used in the reaction . Careful control of these factors is important for optimizing the performance of this catalyst .
Propriétés
IUPAC Name |
acetic acid;dicyclohexylazanide;palladium(2+) | |
---|---|---|
Source | PubChem | |
URL | https://pubchem.ncbi.nlm.nih.gov | |
Description | Data deposited in or computed by PubChem | |
InChI |
InChI=1S/2C12H22N.2C2H4O2.Pd/c2*1-3-7-11(8-4-1)13-12-9-5-2-6-10-12;2*1-2(3)4;/h2*11-12H,1-10H2;2*1H3,(H,3,4);/q2*-1;;;+2 | |
Source | PubChem | |
URL | https://pubchem.ncbi.nlm.nih.gov | |
Description | Data deposited in or computed by PubChem | |
InChI Key |
LAYDWGNLLRXNPH-UHFFFAOYSA-N | |
Source | PubChem | |
URL | https://pubchem.ncbi.nlm.nih.gov | |
Description | Data deposited in or computed by PubChem | |
Canonical SMILES |
CC(=O)O.CC(=O)O.C1CCC(CC1)[N-]C2CCCCC2.C1CCC(CC1)[N-]C2CCCCC2.[Pd+2] | |
Source | PubChem | |
URL | https://pubchem.ncbi.nlm.nih.gov | |
Description | Data deposited in or computed by PubChem | |
Molecular Formula |
C28H52N2O4Pd | |
Source | PubChem | |
URL | https://pubchem.ncbi.nlm.nih.gov | |
Description | Data deposited in or computed by PubChem | |
Molecular Weight |
587.1 g/mol | |
Source | PubChem | |
URL | https://pubchem.ncbi.nlm.nih.gov | |
Description | Data deposited in or computed by PubChem | |
Retrosynthesis Analysis
AI-Powered Synthesis Planning: Our tool employs the Template_relevance Pistachio, Template_relevance Bkms_metabolic, Template_relevance Pistachio_ringbreaker, Template_relevance Reaxys, Template_relevance Reaxys_biocatalysis model, leveraging a vast database of chemical reactions to predict feasible synthetic routes.
One-Step Synthesis Focus: Specifically designed for one-step synthesis, it provides concise and direct routes for your target compounds, streamlining the synthesis process.
Accurate Predictions: Utilizing the extensive PISTACHIO, BKMS_METABOLIC, PISTACHIO_RINGBREAKER, REAXYS, REAXYS_BIOCATALYSIS database, our tool offers high-accuracy predictions, reflecting the latest in chemical research and data.
Strategy Settings
Precursor scoring | Relevance Heuristic |
---|---|
Min. plausibility | 0.01 |
Model | Template_relevance |
Template Set | Pistachio/Bkms_metabolic/Pistachio_ringbreaker/Reaxys/Reaxys_biocatalysis |
Top-N result to add to graph | 6 |
Feasible Synthetic Routes
A: Although the provided research article [] does not specifically utilize trans-Bis(dicyclohexylamine)palladium(II) acetate, this palladium complex is known to be a useful catalyst in various cross-coupling reactions, such as Buchwald-Hartwig aminations. Given that the synthesis of 6-thienyl-substituted 2-amino-3-cyanopyridines likely involves the formation of a carbon-nitrogen bond, exploring the use of trans-Bis(dicyclohexylamine)palladium(II) acetate or similar palladium catalysts could be a potential avenue for optimizing the synthesis route. Further research would be needed to determine the specific reaction conditions and effectiveness of this catalyst in this particular reaction.
Avertissement et informations sur les produits de recherche in vitro
Veuillez noter que tous les articles et informations sur les produits présentés sur BenchChem sont destinés uniquement à des fins informatives. Les produits disponibles à l'achat sur BenchChem sont spécifiquement conçus pour des études in vitro, qui sont réalisées en dehors des organismes vivants. Les études in vitro, dérivées du terme latin "in verre", impliquent des expériences réalisées dans des environnements de laboratoire contrôlés à l'aide de cellules ou de tissus. Il est important de noter que ces produits ne sont pas classés comme médicaments et n'ont pas reçu l'approbation de la FDA pour la prévention, le traitement ou la guérison de toute condition médicale, affection ou maladie. Nous devons souligner que toute forme d'introduction corporelle de ces produits chez les humains ou les animaux est strictement interdite par la loi. Il est essentiel de respecter ces directives pour assurer la conformité aux normes légales et éthiques en matière de recherche et d'expérimentation.