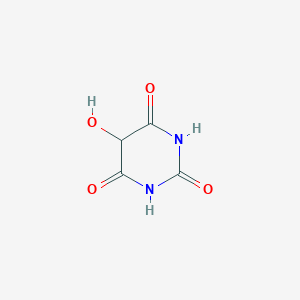
Dialuric acid
Vue d'ensemble
Description
Dialuric acid, also known as 5-hydroxybarbituric acid, is an organic compound that plays a significant role in various biochemical processes. It is a reduction product of alloxan and is known for its involvement in redox reactions, particularly in the context of diabetes research. This compound is a white crystalline solid that is soluble in water and has a molecular formula of C4H4N2O4.
Méthodes De Préparation
Synthetic Routes and Reaction Conditions: Dialuric acid can be synthesized through the reduction of alloxan One common method involves the use of hydrogen sulfide as a reducing agent The reaction is typically carried out in an aqueous solution under nitrogen atmosphere to prevent oxidationThe resulting solution is then washed with carbon disulfide to remove impurities, and the this compound is crystallized out .
Industrial Production Methods: While there is limited information on the large-scale industrial production of this compound, the laboratory synthesis methods can be scaled up with appropriate modifications to ensure purity and yield. The use of hydrogen sulfide and nitrogen atmosphere would be critical in preventing unwanted side reactions and ensuring the stability of the product.
Analyse Des Réactions Chimiques
Autoxidation and Reactive Oxygen Species (ROS) Generation
Dialuric acid undergoes spontaneous autoxidation in aqueous solutions, producing superoxide radicals () and hydrogen peroxide () via a chain reaction mechanism .
Key Reaction Pathways:
-
Primary oxidation :
-
Secondary reactions :
Experimental Data:
Parameter | Value (pH 7.4, 37°C) | Source |
---|---|---|
Initial oxidation rate | ||
Lag period (100 μM) | ~15 minutes | |
yield | 0.8–1.2 equivalents |
Catalysts/Inhibitors :
Metal-Catalyzed Hydroxyl Radical Formation
In the presence of iron salts, this compound generates hydroxyl radicals () via Fenton-like reactions :
Reaction Mechanism:
Kinetic Parameters:
Rate Constant | Value () | Source |
---|---|---|
Biological Implications :
Redox Cycling with Alloxan
This compound participates in reversible redox cycling with its oxidized form, alloxan :
Equilibrium Reactions:
Redox Potentials:
Redox Pair | (mV vs. SHE) | Source |
---|---|---|
Key Observations :
-
The radical intermediate () disproportionates rapidly:
-
Sustained cycling amplifies ROS production, exacerbating oxidative stress .
Reactivity with Biological Thiols
This compound reacts with glutathione (GSH) and other thiols, forming mixed disulfides and depleting cellular antioxidants:
Reaction Pathway:
Impact :
Applications De Recherche Scientifique
Biochemical Research
Dialuric acid is primarily studied for its involvement in redox reactions and its role as a reactive oxygen species (ROS) generator. Its autoxidation process has been shown to produce superoxide radicals and hydrogen peroxide, which are significant in understanding oxidative stress mechanisms in cells.
Autoxidation Studies
Research indicates that the autoxidation of this compound is catalyzed by transition metals such as copper, iron, and manganese. This reaction is crucial in the context of diabetes research, particularly concerning the diabetogenic effects of alloxan. The formation of hydroxyl radicals during this process is particularly noteworthy, as it highlights potential pathways for cellular damage and disease progression .
Toxicological Implications
This compound's role in toxicology is primarily linked to its interaction with biological systems under oxidative stress conditions. It serves as a model compound for studying the effects of ROS on cellular components.
Hemolysis Assays
In veterinary clinical nutrition, this compound has been utilized in hemolysis assays to evaluate the oxidative stress response in animal models. Although not widely available for clinical use, these assays provide insights into dietary impacts on oxidative damage in animals .
Redox Chemistry
The redox properties of this compound have been extensively investigated, particularly its cyclic relationship with alloxan. This redox cycle is fundamental for understanding how this compound can influence cellular environments and contribute to oxidative stress-related diseases.
Research Findings
Studies have demonstrated that the reduction of alloxan to this compound and its subsequent re-oxidation can lead to the generation of superoxide radicals. This cycle is vital for exploring therapeutic approaches to mitigate oxidative damage in diabetic conditions .
Potential Therapeutic Applications
Given its properties, this compound may have potential therapeutic applications, especially in managing conditions associated with oxidative stress.
Diabetes Research
This compound's involvement in diabetic retinopathy models indicates its potential as a biomarker or therapeutic target for diabetes management. Understanding its role could lead to novel strategies for preventing or treating diabetic complications .
Summary Table of Applications
Application Area | Description | Key Findings/Implications |
---|---|---|
Biochemical Research | Study of autoxidation and ROS generation | Catalyzed by metals; significant in diabetes |
Toxicology | Hemolysis assays for oxidative stress evaluation | Insights into dietary impacts on health |
Redox Chemistry | Investigation of alloxan-dialuric acid redox cycle | Important for understanding oxidative damage |
Therapeutics | Potential role in diabetes management | May serve as a biomarker or treatment target |
Mécanisme D'action
Dialuric acid exerts its effects primarily through its involvement in redox reactions. In the presence of intracellular thiols such as glutathione, this compound undergoes autoxidation, generating superoxide radicals, hydrogen peroxide, and hydroxyl radicals. These ROS are highly reactive and can cause significant cellular damage. In the context of diabetes research, the ROS generated by this compound contribute to the destruction of pancreatic beta cells, leading to insulin-dependent diabetes .
Comparaison Avec Des Composés Similaires
Dialuric acid is closely related to alloxan, both of which are involved in redox cycling and the generation of ROS. Other similar compounds include:
Alloxantin: A molecular compound of alloxan and this compound, which also participates in redox reactions.
Streptozotocin: Another diabetogenic agent that, like alloxan, induces diabetes by generating ROS and causing beta-cell destruction.
Uniqueness: this compound’s unique role in redox cycling and its ability to generate a variety of ROS make it a valuable compound in research focused on oxidative stress and diabetes. Its specific interactions with intracellular thiols and its involvement in the autoxidation process distinguish it from other similar compounds.
Activité Biologique
Dialuric acid, a derivative of uric acid, has garnered attention for its biological activities, particularly in relation to oxidative stress and its bactericidal properties. This article explores the mechanisms through which this compound exerts its effects, supported by various research findings, case studies, and data tables.
This compound is known for its ability to generate reactive oxygen species (ROS), including superoxide anions and hydrogen peroxide (H2O2). These species play a crucial role in the compound's biological activity.
- Auto-oxidation : this compound undergoes auto-oxidation, producing H2O2 through an O2-dependent chain mechanism. This process is significantly influenced by pH, temperature, and the concentration of this compound itself. The oxidation rate varies, with notable differences observed among related compounds such as divicine and isouramil .
- Bactericidal Activity : Research indicates that this compound exhibits bactericidal properties against Escherichia coli and Staphylococcus aureus. The inhibition of bacterial growth is dose-dependent and primarily attributed to the accumulation of H2O2 rather than superoxide radicals. The addition of catalase can reverse this inhibition, highlighting the role of H2O2 in the bactericidal mechanism .
Effects on Cellular Metabolism
This compound's interaction with human neutrophils demonstrates its impact on cellular metabolism:
- Hexose Monophosphate Shunt Activation : The addition of this compound to resting human neutrophils stimulates the hexose monophosphate shunt, a metabolic pathway crucial for generating NADPH and ribose-5-phosphate. This activation correlates with increased oxygen consumption and suggests a role in enhancing the oxidative burst necessary for microbial killing .
Toxicological Implications
While this compound has beneficial bactericidal properties, it also poses toxicity risks, particularly to pancreatic beta cells. The compound's auto-oxidation leads to oxidative stress, which can damage cellular structures:
- Toxicity Mechanisms : The toxicity observed in pancreatic beta cells is linked to redox cycling and the generation of ROS. Studies have shown that intracellular superoxide dismutase provides only temporary protection against this compound's oxidative effects, necessitating further investigation into protective strategies against its toxic actions .
Case Studies and Research Findings
Several studies have elucidated the biological activity of this compound:
- Bactericidal Studies : A study demonstrated that this compound inhibited bacterial growth in vitro, with a clear dose-response relationship observed. The mechanism was primarily through H2O2 generation rather than direct action on bacterial cells .
- Toxicity in Beta Cells : Research indicated that this compound induces oxidative stress in pancreatic beta cells, contributing to cell death. This effect is exacerbated by the presence of transition metals that catalyze its auto-oxidation .
- Redox Cycling Investigations : Theoretical investigations into the alloxan-dialuric acid redox cycle have provided insights into how these compounds interact at a molecular level, influencing their biological activity and potential therapeutic applications .
Data Tables
The following table summarizes key findings related to the biological activity of this compound:
Propriétés
IUPAC Name |
5-hydroxy-1,3-diazinane-2,4,6-trione | |
---|---|---|
Source | PubChem | |
URL | https://pubchem.ncbi.nlm.nih.gov | |
Description | Data deposited in or computed by PubChem | |
InChI |
InChI=1S/C4H4N2O4/c7-1-2(8)5-4(10)6-3(1)9/h1,7H,(H2,5,6,8,9,10) | |
Source | PubChem | |
URL | https://pubchem.ncbi.nlm.nih.gov | |
Description | Data deposited in or computed by PubChem | |
InChI Key |
BVEWMNTVZPFPQI-UHFFFAOYSA-N | |
Source | PubChem | |
URL | https://pubchem.ncbi.nlm.nih.gov | |
Description | Data deposited in or computed by PubChem | |
Canonical SMILES |
C1(C(=O)NC(=O)NC1=O)O | |
Source | PubChem | |
URL | https://pubchem.ncbi.nlm.nih.gov | |
Description | Data deposited in or computed by PubChem | |
Molecular Formula |
C4H4N2O4 | |
Source | PubChem | |
URL | https://pubchem.ncbi.nlm.nih.gov | |
Description | Data deposited in or computed by PubChem | |
DSSTOX Substance ID |
DTXSID601265223 | |
Record name | 5-Hydroxy-2,4,6(1H,3H,5H)-pyrimidinetrione | |
Source | EPA DSSTox | |
URL | https://comptox.epa.gov/dashboard/DTXSID601265223 | |
Description | DSSTox provides a high quality public chemistry resource for supporting improved predictive toxicology. | |
Molecular Weight |
144.09 g/mol | |
Source | PubChem | |
URL | https://pubchem.ncbi.nlm.nih.gov | |
Description | Data deposited in or computed by PubChem | |
CAS No. |
444-15-5 | |
Record name | 5-Hydroxy-2,4,6(1H,3H,5H)-pyrimidinetrione | |
Source | CAS Common Chemistry | |
URL | https://commonchemistry.cas.org/detail?cas_rn=444-15-5 | |
Description | CAS Common Chemistry is an open community resource for accessing chemical information. Nearly 500,000 chemical substances from CAS REGISTRY cover areas of community interest, including common and frequently regulated chemicals, and those relevant to high school and undergraduate chemistry classes. This chemical information, curated by our expert scientists, is provided in alignment with our mission as a division of the American Chemical Society. | |
Explanation | The data from CAS Common Chemistry is provided under a CC-BY-NC 4.0 license, unless otherwise stated. | |
Record name | 5-Hydroxy-2,4,6(1H,3H,5H)-pyrimidinetrione | |
Source | EPA DSSTox | |
URL | https://comptox.epa.gov/dashboard/DTXSID601265223 | |
Description | DSSTox provides a high quality public chemistry resource for supporting improved predictive toxicology. | |
Record name | 5-hydroxybarbituric acid | |
Source | European Chemicals Agency (ECHA) | |
URL | https://echa.europa.eu/substance-information/-/substanceinfo/100.006.497 | |
Description | The European Chemicals Agency (ECHA) is an agency of the European Union which is the driving force among regulatory authorities in implementing the EU's groundbreaking chemicals legislation for the benefit of human health and the environment as well as for innovation and competitiveness. | |
Explanation | Use of the information, documents and data from the ECHA website is subject to the terms and conditions of this Legal Notice, and subject to other binding limitations provided for under applicable law, the information, documents and data made available on the ECHA website may be reproduced, distributed and/or used, totally or in part, for non-commercial purposes provided that ECHA is acknowledged as the source: "Source: European Chemicals Agency, http://echa.europa.eu/". Such acknowledgement must be included in each copy of the material. ECHA permits and encourages organisations and individuals to create links to the ECHA website under the following cumulative conditions: Links can only be made to webpages that provide a link to the Legal Notice page. | |
Record name | Dialuric acid | |
Source | FDA Global Substance Registration System (GSRS) | |
URL | https://gsrs.ncats.nih.gov/ginas/app/beta/substances/T856T7DXN9 | |
Description | The FDA Global Substance Registration System (GSRS) enables the efficient and accurate exchange of information on what substances are in regulated products. Instead of relying on names, which vary across regulatory domains, countries, and regions, the GSRS knowledge base makes it possible for substances to be defined by standardized, scientific descriptions. | |
Explanation | Unless otherwise noted, the contents of the FDA website (www.fda.gov), both text and graphics, are not copyrighted. They are in the public domain and may be republished, reprinted and otherwise used freely by anyone without the need to obtain permission from FDA. Credit to the U.S. Food and Drug Administration as the source is appreciated but not required. | |
Retrosynthesis Analysis
AI-Powered Synthesis Planning: Our tool employs the Template_relevance Pistachio, Template_relevance Bkms_metabolic, Template_relevance Pistachio_ringbreaker, Template_relevance Reaxys, Template_relevance Reaxys_biocatalysis model, leveraging a vast database of chemical reactions to predict feasible synthetic routes.
One-Step Synthesis Focus: Specifically designed for one-step synthesis, it provides concise and direct routes for your target compounds, streamlining the synthesis process.
Accurate Predictions: Utilizing the extensive PISTACHIO, BKMS_METABOLIC, PISTACHIO_RINGBREAKER, REAXYS, REAXYS_BIOCATALYSIS database, our tool offers high-accuracy predictions, reflecting the latest in chemical research and data.
Strategy Settings
Precursor scoring | Relevance Heuristic |
---|---|
Min. plausibility | 0.01 |
Model | Template_relevance |
Template Set | Pistachio/Bkms_metabolic/Pistachio_ringbreaker/Reaxys/Reaxys_biocatalysis |
Top-N result to add to graph | 6 |
Feasible Synthetic Routes
Q1: How does dialuric acid interact with biological systems?
A1: this compound readily undergoes autoxidation in the presence of oxygen [, , , , , ]. This process generates reactive oxygen species (ROS) such as superoxide radicals, hydrogen peroxide, and hydroxyl radicals [, , , , , , , , ]. These ROS can cause oxidative damage to various cellular components, including lipids, proteins, and DNA [, , , , , , , , ], leading to cytotoxicity [, , , , , , ].
Q2: What is the role of glutathione in this compound-induced toxicity?
A2: While glutathione can initially reduce alloxan to this compound, it also participates in a redox cycle with this compound, further promoting ROS generation and exacerbating oxidative stress [, , , ].
Q3: How does this compound affect pancreatic beta cells?
A3: this compound, along with alloxan, contributes to the destruction of pancreatic beta cells, leading to experimental diabetes [, , , , , , , ]. This effect is primarily attributed to the generation of ROS, particularly in the presence of intracellular thiols like glutathione [, , , , , ].
Q4: What is the molecular formula and weight of this compound?
A4: The molecular formula of this compound is C4H4N2O4, and its molecular weight is 144.09 g/mol.
Q5: Is there spectroscopic data available for this compound?
A5: Yes, studies have investigated the proton magnetic resonance (1H NMR) of this compound, particularly regarding its tautomeric forms [].
Q6: How does the presence of water affect this compound?
A6: this compound exists as a monohydrate in its crystalline form []. Desolvation of these crystals can occur, but the resulting anhydrous form is still susceptible to oxidation, especially in humid environments []. High humidity accelerates the solid-state oxidation of this compound to alloxantin [].
Q7: Have there been any computational studies on this compound?
A8: Yes, density functional theory (DFT) has been used to investigate the redox cycle between alloxan and this compound []. This study provided insights into the thermodynamics of the electron and proton transfer steps involved in the cycle [].
Q8: How does the structure of this compound relate to its reactivity?
A9: The presence of the enediol group in this compound makes it susceptible to oxidation [, ]. This structural feature is essential for its participation in redox reactions and the generation of ROS [, ].
Q9: How can the stability of this compound be enhanced?
A10: this compound is inherently unstable due to its susceptibility to oxidation [, , , , , ]. Strategies to enhance its stability often involve minimizing exposure to oxygen and controlling environmental factors such as humidity [].
Avertissement et informations sur les produits de recherche in vitro
Veuillez noter que tous les articles et informations sur les produits présentés sur BenchChem sont destinés uniquement à des fins informatives. Les produits disponibles à l'achat sur BenchChem sont spécifiquement conçus pour des études in vitro, qui sont réalisées en dehors des organismes vivants. Les études in vitro, dérivées du terme latin "in verre", impliquent des expériences réalisées dans des environnements de laboratoire contrôlés à l'aide de cellules ou de tissus. Il est important de noter que ces produits ne sont pas classés comme médicaments et n'ont pas reçu l'approbation de la FDA pour la prévention, le traitement ou la guérison de toute condition médicale, affection ou maladie. Nous devons souligner que toute forme d'introduction corporelle de ces produits chez les humains ou les animaux est strictement interdite par la loi. Il est essentiel de respecter ces directives pour assurer la conformité aux normes légales et éthiques en matière de recherche et d'expérimentation.