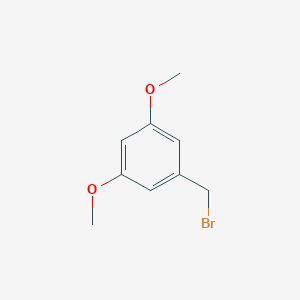
3,5-Dimethoxybenzyl bromide
Vue d'ensemble
Description
3,5-Dimethoxybenzyl bromide is an aromatic bromide . It may be used in the synthesis of various compounds such as 3,4,3′,5′-tetramethoxystilbene, 3,5-dimethoxyphenylacetic acid, and 1-(3,5-dimethoxyphenyl)-4-pentene .
Synthesis Analysis
The compound 1-(bromomethyl)-3,5-dimethoxybenzene was produced in two phases using a technique indicated in a previously reported method . It may also be used as a starting material in the multi-step synthesis of resveratrol and 3,5-dimethoxyhomophthalic acid .Molecular Structure Analysis
The molecular formula of 3,5-Dimethoxybenzyl bromide is C9H11BrO2 and its molecular weight is 231.09 . The structure determination of 3,5-dimethoxybenzyl bromide has been carried out from laboratory powder X-ray diffraction data using the direct-space Genetic Algorithm technique for structure solution followed by Rietveld refinement .Chemical Reactions Analysis
3,5-Dimethoxybenzyl bromide may be used in the synthesis of various compounds such as 3,4,3′,5′-tetramethoxystilbene, 3,5-dimethoxyphenylacetic acid, and 1-(3,5-dimethoxyphenyl)-4-pentene .Physical And Chemical Properties Analysis
3,5-Dimethoxybenzyl bromide is a solid at 20°C . It has a melting point of 69-70°C . It should be stored at a temperature between 0-10°C . It is heat sensitive .Applications De Recherche Scientifique
- 3,4,3′,5′-Tetramethoxystilbene : 3,5-Dimethoxybenzyl bromide serves as a precursor in the synthesis of this stilbene derivative, which exhibits interesting biological properties .
- Resveratrol : It is used as an intermediate in the multi-step synthesis of resveratrol, a natural polyphenol found in red grapes and known for its potential health benefits .
- 3,5-Dimethoxyphenylacetic Acid : The compound participates in the synthesis of this phenylacetic acid derivative, which may have applications in pharmaceutical research .
- 1-(3,5-Dimethoxyphenyl)-4-pentene : 3,5-Dimethoxybenzyl bromide is involved in the preparation of this alkene, which can be further functionalized for various purposes .
Stilbene Derivatives Synthesis
Phenylacetic Acid Derivatives
Alkene Synthesis
Other Applications
Safety And Hazards
3,5-Dimethoxybenzyl bromide is classified as a skin corrosive (Category 1B) and causes serious eye damage (Category 1) . It is advised to avoid breathing dust, to wear protective clothing, and to handle it in a well-ventilated area . In case of contact with skin or eyes, it is recommended to rinse with water and seek medical attention .
Relevant Papers The paper “Structural properties of methoxy derivatives of benzyl bromide” discusses the structure determination of 3,5-dimethoxybenzyl bromide and its potential use as building blocks for the synthesis of dendritic materials .
Propriétés
IUPAC Name |
1-(bromomethyl)-3,5-dimethoxybenzene | |
---|---|---|
Source | PubChem | |
URL | https://pubchem.ncbi.nlm.nih.gov | |
Description | Data deposited in or computed by PubChem | |
InChI |
InChI=1S/C9H11BrO2/c1-11-8-3-7(6-10)4-9(5-8)12-2/h3-5H,6H2,1-2H3 | |
Source | PubChem | |
URL | https://pubchem.ncbi.nlm.nih.gov | |
Description | Data deposited in or computed by PubChem | |
InChI Key |
BTHIGJGJAPYFSJ-UHFFFAOYSA-N | |
Source | PubChem | |
URL | https://pubchem.ncbi.nlm.nih.gov | |
Description | Data deposited in or computed by PubChem | |
Canonical SMILES |
COC1=CC(=CC(=C1)CBr)OC | |
Source | PubChem | |
URL | https://pubchem.ncbi.nlm.nih.gov | |
Description | Data deposited in or computed by PubChem | |
Molecular Formula |
C9H11BrO2 | |
Source | PubChem | |
URL | https://pubchem.ncbi.nlm.nih.gov | |
Description | Data deposited in or computed by PubChem | |
DSSTOX Substance ID |
DTXSID20361564 | |
Record name | 3,5-Dimethoxybenzyl bromide | |
Source | EPA DSSTox | |
URL | https://comptox.epa.gov/dashboard/DTXSID20361564 | |
Description | DSSTox provides a high quality public chemistry resource for supporting improved predictive toxicology. | |
Molecular Weight |
231.09 g/mol | |
Source | PubChem | |
URL | https://pubchem.ncbi.nlm.nih.gov | |
Description | Data deposited in or computed by PubChem | |
Product Name |
3,5-Dimethoxybenzyl bromide | |
CAS RN |
877-88-3 | |
Record name | 3,5-Dimethoxybenzyl bromide | |
Source | CAS Common Chemistry | |
URL | https://commonchemistry.cas.org/detail?cas_rn=877-88-3 | |
Description | CAS Common Chemistry is an open community resource for accessing chemical information. Nearly 500,000 chemical substances from CAS REGISTRY cover areas of community interest, including common and frequently regulated chemicals, and those relevant to high school and undergraduate chemistry classes. This chemical information, curated by our expert scientists, is provided in alignment with our mission as a division of the American Chemical Society. | |
Explanation | The data from CAS Common Chemistry is provided under a CC-BY-NC 4.0 license, unless otherwise stated. | |
Record name | 3,5-Dimethoxybenzyl bromide | |
Source | EPA DSSTox | |
URL | https://comptox.epa.gov/dashboard/DTXSID20361564 | |
Description | DSSTox provides a high quality public chemistry resource for supporting improved predictive toxicology. | |
Synthesis routes and methods I
Procedure details
Synthesis routes and methods II
Procedure details
Synthesis routes and methods III
Procedure details
Synthesis routes and methods IV
Procedure details
Retrosynthesis Analysis
AI-Powered Synthesis Planning: Our tool employs the Template_relevance Pistachio, Template_relevance Bkms_metabolic, Template_relevance Pistachio_ringbreaker, Template_relevance Reaxys, Template_relevance Reaxys_biocatalysis model, leveraging a vast database of chemical reactions to predict feasible synthetic routes.
One-Step Synthesis Focus: Specifically designed for one-step synthesis, it provides concise and direct routes for your target compounds, streamlining the synthesis process.
Accurate Predictions: Utilizing the extensive PISTACHIO, BKMS_METABOLIC, PISTACHIO_RINGBREAKER, REAXYS, REAXYS_BIOCATALYSIS database, our tool offers high-accuracy predictions, reflecting the latest in chemical research and data.
Strategy Settings
Precursor scoring | Relevance Heuristic |
---|---|
Min. plausibility | 0.01 |
Model | Template_relevance |
Template Set | Pistachio/Bkms_metabolic/Pistachio_ringbreaker/Reaxys/Reaxys_biocatalysis |
Top-N result to add to graph | 6 |
Feasible Synthetic Routes
Q & A
Q1: What is a common synthetic route to produce 3,5-Dimethoxybenzyl bromide?
A1: A two-step synthesis demonstrates efficiency in producing 3,5-Dimethoxybenzyl bromide []. First, 3,5-dimethoxybenzoic acid undergoes reduction using Sodium borohydride (NaBH4) and Iodine (I2) to yield 3,5-dimethoxybenzyl alcohol. Subsequently, bromination with 40% Hydrobromic acid (HBr) yields the desired 3,5-Dimethoxybenzyl bromide with an overall yield of 70%. []
Q2: How does the structure of 3,5-Dimethoxybenzyl bromide relate to its applications in synthesizing complex molecules?
A2: The presence of the bromide group allows for facile substitution reactions, making it a valuable precursor for various compounds. For instance, it acts as a starting material in the synthesis of novel stilbenes by reacting with sodium cyanide, undergoing subsequent hydrolysis, condensation, and demethylation. [] Additionally, it enables the synthesis of deuterated and tritiated forms of Δ8 and Δ9 - tetrahydrocannabinol (THC). This involves a cross-coupling reaction with 4-buten-magnesium bromide in the presence of Lithium di-copper tetrachloride (Li2CuCl4) to yield 1-(3,5-dimethoxyphenyl)-4-pentene. Further reactions eventually lead to the desired THC analogs. []
Q3: Has the crystal structure of 3,5-Dimethoxybenzyl bromide been determined, and if so, what insights does it provide?
A3: Yes, the crystal structures of both 3,5-dimethoxybenzyl bromide and its close analog, 3,4,5-trimethoxybenzyl bromide, have been determined using powder X-ray diffraction data. [] Interestingly, despite the seemingly minor structural difference of a single methoxy group at the 4-position, the two compounds exhibit significant differences in their structural properties. This highlights the impact of even small changes in molecular structure on the overall solid-state arrangement and potentially on their reactivity and applications. []
Q4: Are there examples of 3,5-Dimethoxybenzyl bromide being used to synthesize complex supramolecular structures?
A4: Yes, researchers have successfully employed 3,5-Dimethoxybenzyl bromide in the construction of rotaxanes. By reacting 3,5-Dimethoxybenzyl bromide with an alpha-cyclodextrin (α-CD) complex of 1-(N-carbazole)-10-[4-(4-pyridinio)-1-pyridinio]decane in dimethylformamide (DMF), two isomeric []rotaxanes (2a and 2b) are formed. This highlights the ability of 3,5-Dimethoxybenzyl bromide to participate in reactions leading to complex molecular architectures with potential applications in materials science and nanotechnology. []
Q5: Beyond its use in material synthesis, has 3,5-Dimethoxybenzyl bromide been explored for its biological activity?
A5: Indeed, studies have investigated the photolysis of 3,5-Dimethoxybenzyl bromide and related compounds (chloride, iodide, and acetate) in various alcohols like methanol and tert-butyl alcohol. [] These studies shed light on the photochemical behavior of these benzyl derivatives and their potential reactivity in biological systems, particularly in the presence of light. While this specific research focuses on photochemical mechanisms, it provides a starting point for exploring the potential biological activities of 3,5-Dimethoxybenzyl bromide and its derivatives.
Q6: Are there any applications of 3,5-Dimethoxybenzyl bromide in the development of new antibacterial agents?
A6: Research indicates the potential of 3,5-dimethoxystilbene derivatives, synthesized using 3,5-Dimethoxybenzyl bromide as a precursor, as potential antibacterial agents. [] These derivatives show promising activity against drug-resistant bacteria, highlighting their importance in combating the growing threat of antimicrobial resistance. The synthesis involves a Wittig-Honner reaction of 3,5-dimethoxybenzyl phosphonate (derived from 3,5-Dimethoxybenzyl bromide) with an appropriate aldehyde. [] This avenue of research holds promise for developing new strategies to address the global challenge of drug-resistant infections.
Q7: Can 3,5-Dimethoxybenzyl bromide be used to synthesize natural products or their analogs?
A7: Yes, 3,5-Dimethoxybenzyl bromide plays a key role in efficiently synthesizing 3,5-dimethoxyhomophthalic acid, a crucial intermediate in the preparation of natural isocoumarins. [] This synthesis proceeds through a rhodium-catalyzed carbonylation of 3,5-Dimethoxybenzyl bromide to produce 3,5-dimethoxyphenylacetic acid, followed by o-formylation and oxidation to yield the desired 3,5-dimethoxyhomophthalic acid. [] This compound can then be used to synthesize various isocoumarins, including those structurally related to natural products like agrimonolide and achlisocoumarin 1. [] This highlights the utility of 3,5-Dimethoxybenzyl bromide in accessing valuable natural product-like scaffolds for potential medicinal chemistry applications.
Avertissement et informations sur les produits de recherche in vitro
Veuillez noter que tous les articles et informations sur les produits présentés sur BenchChem sont destinés uniquement à des fins informatives. Les produits disponibles à l'achat sur BenchChem sont spécifiquement conçus pour des études in vitro, qui sont réalisées en dehors des organismes vivants. Les études in vitro, dérivées du terme latin "in verre", impliquent des expériences réalisées dans des environnements de laboratoire contrôlés à l'aide de cellules ou de tissus. Il est important de noter que ces produits ne sont pas classés comme médicaments et n'ont pas reçu l'approbation de la FDA pour la prévention, le traitement ou la guérison de toute condition médicale, affection ou maladie. Nous devons souligner que toute forme d'introduction corporelle de ces produits chez les humains ou les animaux est strictement interdite par la loi. Il est essentiel de respecter ces directives pour assurer la conformité aux normes légales et éthiques en matière de recherche et d'expérimentation.