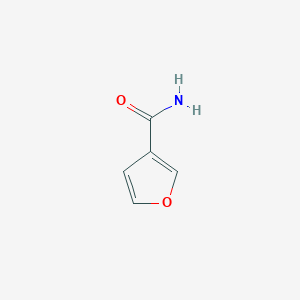
Furan-3-carboxamide
Vue d'ensemble
Description
Furan-3-carboxamide is a chemical compound with the CAS Number: 609-35-8 . It has a molecular weight of 111.1 and its IUPAC name is 3-furamide .
Synthesis Analysis
Furan-carboxamide derivatives have been synthesized from acyl chlorides and heterocyclic amine derivatives . The target products were obtained in one step through a simple coupling reaction of these two components, yielding the furan-carboxamide derivatives in 30–80% yields .Molecular Structure Analysis
The molecular structure of Furan-3-carboxamide was confirmed using different spectroscopic methods including IR, 1H NMR, 13C NMR, and elemental analysis .Chemical Reactions Analysis
Furan-carboxamide derivatives have been used as model compounds to study the competition between two modes of indirect photochemistry . They have been used to investigate structure−reactivity relationships in photodegradation reactions involving singlet oxygen (1O2) and triplet chromophoric dissolved organic matter (3CDOM*) .Physical And Chemical Properties Analysis
Furan-3-carboxamide is a solid at room temperature . It has a boiling point of 274.6±13.0 C at 760 mmHg and a melting point of 169-171 C .Applications De Recherche Scientifique
Synthesis and Antimicrobial Activity
- Antimicrobial Properties : Furan-3-carboxamides have shown significant in vitro antimicrobial activity against yeast, filamentous fungi, bacteria, and algae. This makes them promising candidates for developing new antimicrobial agents (Zanatta et al., 2007).
Chemical Reactivity and Biological Applications
- Chemical and Biological Applications : Furan-carboxamides exhibit variable and important biological properties. They have been used in synthesizing different heterocycles and have applications in reactions and biological processes (Monier et al., 2018).
Influenza A Virus Inhibition
- Influenza A Virus Inhibition : Certain furan-carboxamide derivatives are potent inhibitors of the H5N1 influenza A virus. Their structure-activity relationship studies reveal the importance of specific substitutions for antiviral activity (Yongshi et al., 2017).
Synthesis Techniques
- Novel Synthesis Methods : Innovative synthesis methods for furan-3-carboxamides have been developed, enabling the creation of diverse and potentially biologically active molecules (Sultana et al., 2018).
Antitumor Properties
- Antitumor Applications : Furan-carboxamides, specifically anthra[2,3-b]furan-3-carboxamides, exhibit cytotoxicity towards tumor cells, including those resistant to certain drugs. They show promise in overcoming drug resistance in cancer treatment (Volodina et al., 2019).
Molecular Recognition of DNA
- DNA Binding : Furan amino acids, such as those found in furan carboxamides, have been incorporated into DNA-binding agents, demonstrating effective stabilization of duplex DNA and potential for molecular recognition applications (Muzikar et al., 2011).
Mécanisme D'action
Target of Action
Furan-3-carboxamide is a furan derivative that has been found to exhibit a wide range of biological and pharmacological characteristics Furan derivatives have been employed as medicines in a number of distinct disease areas , suggesting a broad spectrum of potential targets.
Mode of Action
Furan derivatives are known for their reactivity . They have been used in the synthesis of numerous innovative antibacterial agents , indicating that they may interact with their targets to inhibit bacterial growth
Biochemical Pathways
Furan-containing compounds are known to exhibit a wide range of advantageous biological and pharmacological characteristics , suggesting that they may influence multiple biochemical pathways
Result of Action
Furan derivatives have been employed as medicines in a number of distinct disease areas , suggesting that they may have a variety of molecular and cellular effects
Safety and Hazards
Orientations Futures
Propriétés
IUPAC Name |
furan-3-carboxamide | |
---|---|---|
Source | PubChem | |
URL | https://pubchem.ncbi.nlm.nih.gov | |
Description | Data deposited in or computed by PubChem | |
InChI |
InChI=1S/C5H5NO2/c6-5(7)4-1-2-8-3-4/h1-3H,(H2,6,7) | |
Source | PubChem | |
URL | https://pubchem.ncbi.nlm.nih.gov | |
Description | Data deposited in or computed by PubChem | |
InChI Key |
GOLHZPOXCLTFRB-UHFFFAOYSA-N | |
Source | PubChem | |
URL | https://pubchem.ncbi.nlm.nih.gov | |
Description | Data deposited in or computed by PubChem | |
Canonical SMILES |
C1=COC=C1C(=O)N | |
Source | PubChem | |
URL | https://pubchem.ncbi.nlm.nih.gov | |
Description | Data deposited in or computed by PubChem | |
Molecular Formula |
C5H5NO2 | |
Source | PubChem | |
URL | https://pubchem.ncbi.nlm.nih.gov | |
Description | Data deposited in or computed by PubChem | |
DSSTOX Substance ID |
DTXSID20591586 | |
Record name | Furan-3-carboxamide | |
Source | EPA DSSTox | |
URL | https://comptox.epa.gov/dashboard/DTXSID20591586 | |
Description | DSSTox provides a high quality public chemistry resource for supporting improved predictive toxicology. | |
Molecular Weight |
111.10 g/mol | |
Source | PubChem | |
URL | https://pubchem.ncbi.nlm.nih.gov | |
Description | Data deposited in or computed by PubChem | |
CAS RN |
609-35-8 | |
Record name | 3-Furancarboxamide | |
Source | CAS Common Chemistry | |
URL | https://commonchemistry.cas.org/detail?cas_rn=609-35-8 | |
Description | CAS Common Chemistry is an open community resource for accessing chemical information. Nearly 500,000 chemical substances from CAS REGISTRY cover areas of community interest, including common and frequently regulated chemicals, and those relevant to high school and undergraduate chemistry classes. This chemical information, curated by our expert scientists, is provided in alignment with our mission as a division of the American Chemical Society. | |
Explanation | The data from CAS Common Chemistry is provided under a CC-BY-NC 4.0 license, unless otherwise stated. | |
Record name | Furan-3-carboxamide | |
Source | EPA DSSTox | |
URL | https://comptox.epa.gov/dashboard/DTXSID20591586 | |
Description | DSSTox provides a high quality public chemistry resource for supporting improved predictive toxicology. | |
Retrosynthesis Analysis
AI-Powered Synthesis Planning: Our tool employs the Template_relevance Pistachio, Template_relevance Bkms_metabolic, Template_relevance Pistachio_ringbreaker, Template_relevance Reaxys, Template_relevance Reaxys_biocatalysis model, leveraging a vast database of chemical reactions to predict feasible synthetic routes.
One-Step Synthesis Focus: Specifically designed for one-step synthesis, it provides concise and direct routes for your target compounds, streamlining the synthesis process.
Accurate Predictions: Utilizing the extensive PISTACHIO, BKMS_METABOLIC, PISTACHIO_RINGBREAKER, REAXYS, REAXYS_BIOCATALYSIS database, our tool offers high-accuracy predictions, reflecting the latest in chemical research and data.
Strategy Settings
Precursor scoring | Relevance Heuristic |
---|---|
Min. plausibility | 0.01 |
Model | Template_relevance |
Template Set | Pistachio/Bkms_metabolic/Pistachio_ringbreaker/Reaxys/Reaxys_biocatalysis |
Top-N result to add to graph | 6 |
Feasible Synthetic Routes
Avertissement et informations sur les produits de recherche in vitro
Veuillez noter que tous les articles et informations sur les produits présentés sur BenchChem sont destinés uniquement à des fins informatives. Les produits disponibles à l'achat sur BenchChem sont spécifiquement conçus pour des études in vitro, qui sont réalisées en dehors des organismes vivants. Les études in vitro, dérivées du terme latin "in verre", impliquent des expériences réalisées dans des environnements de laboratoire contrôlés à l'aide de cellules ou de tissus. Il est important de noter que ces produits ne sont pas classés comme médicaments et n'ont pas reçu l'approbation de la FDA pour la prévention, le traitement ou la guérison de toute condition médicale, affection ou maladie. Nous devons souligner que toute forme d'introduction corporelle de ces produits chez les humains ou les animaux est strictement interdite par la loi. Il est essentiel de respecter ces directives pour assurer la conformité aux normes légales et éthiques en matière de recherche et d'expérimentation.