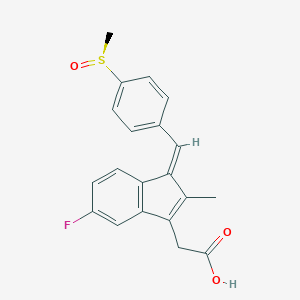
(S)-Sulindac
Vue d'ensemble
Description
(S)-Sulindac is a non-steroidal anti-inflammatory drug (NSAID) that belongs to the class of arylalkanoic acids. It is the (S)-enantiomer of sulindac, which is known for its anti-inflammatory, analgesic, and antipyretic properties. Sulindac is commonly used to treat conditions such as rheumatoid arthritis, osteoarthritis, and ankylosing spondylitis. The (S)-enantiomer is specifically noted for its enhanced pharmacological activity compared to its ®-counterpart.
Méthodes De Préparation
Synthetic Routes and Reaction Conditions: The synthesis of (S)-Sulindac typically involves the resolution of racemic sulindac or the asymmetric synthesis starting from chiral precursors. One common method includes the following steps:
Synthesis of racemic sulindac: This involves the reaction of 2-chlorobenzyl chloride with 4-fluorobenzyl cyanide in the presence of a base to form the intermediate, which is then hydrolyzed to produce racemic sulindac.
Resolution of racemic sulindac: This can be achieved using chiral resolution agents such as tartaric acid derivatives to separate the (S)-enantiomer from the ®-enantiomer.
Industrial Production Methods: Industrial production of this compound often employs large-scale chiral resolution techniques or asymmetric synthesis methods. The use of chiral catalysts and advanced separation technologies ensures the efficient production of the desired enantiomer with high purity.
Analyse Des Réactions Chimiques
Types of Reactions: (S)-Sulindac undergoes various chemical reactions, including:
Oxidation: It can be oxidized to form sulindac sulfone, an inactive metabolite.
Reduction: Reduction of this compound can yield sulindac sulfide, which is the active form of the drug.
Substitution: The aromatic rings in this compound can undergo electrophilic substitution reactions.
Common Reagents and Conditions:
Oxidation: Common oxidizing agents include hydrogen peroxide and potassium permanganate.
Reduction: Reducing agents such as sodium borohydride or catalytic hydrogenation are used.
Substitution: Electrophilic substitution reactions often require catalysts like aluminum chloride or iron(III) chloride.
Major Products:
Sulindac sulfone: Formed through oxidation.
Sulindac sulfide: Formed through reduction.
Applications De Recherche Scientifique
(S)-Sulindac has a wide range of applications in scientific research:
Chemistry: It is used as a model compound in studies of chiral resolution and asymmetric synthesis.
Biology: Research on this compound includes its effects on cellular processes and its potential as a chemopreventive agent.
Medicine: It is studied for its anti-inflammatory and analgesic properties, as well as its potential role in cancer prevention and treatment.
Industry: this compound is used in the development of new NSAIDs and in the study of drug metabolism and pharmacokinetics.
Mécanisme D'action
The mechanism of action of (S)-Sulindac involves the inhibition of cyclooxygenase (COX) enzymes, specifically COX-1 and COX-2. This inhibition reduces the synthesis of prostaglandins, which are mediators of inflammation, pain, and fever. The (S)-enantiomer of sulindac is more effective in inhibiting COX-2, which is primarily responsible for the anti-inflammatory effects. Additionally, this compound has been shown to induce apoptosis in cancer cells through the activation of the mitochondrial pathway and the inhibition of nuclear factor kappa B (NF-κB) signaling.
Comparaison Avec Des Composés Similaires
Ibuprofen: Another NSAID with similar anti-inflammatory and analgesic properties.
Naproxen: An NSAID that also inhibits COX enzymes and is used to treat similar conditions.
Diclofenac: A potent NSAID with a similar mechanism of action.
Uniqueness of (S)-Sulindac:
Chirality: The (S)-enantiomer of sulindac is specifically noted for its enhanced pharmacological activity compared to the ®-enantiomer.
Chemopreventive Potential: this compound has shown promise in cancer prevention and treatment, which is a unique aspect compared to other NSAIDs.
Metabolic Pathways: The distinct metabolic pathways of this compound, including its conversion to active and inactive metabolites, contribute to its unique pharmacokinetic profile.
Activité Biologique
(S)-Sulindac is a nonsteroidal anti-inflammatory drug (NSAID) that has garnered attention not only for its analgesic and anti-inflammatory properties but also for its potential anticancer activity. This article explores the biological activities of this compound, including its mechanisms of action, metabolic pathways, and effects on cancer cells and normal tissues, supported by diverse research findings.
Chemical Structure and Metabolism
This compound is a prodrug that requires metabolic conversion to its active forms: this compound sulfide (SS) and this compound sulfone (SF). The metabolism of this compound is primarily facilitated by liver enzymes and colonic bacteria. The reduction of this compound to SS is catalyzed by methionine sulfoxide reductase (Msr) A, while the oxidation to SF occurs via the cytochrome P450 enzyme system .
Table 1: Metabolic Pathways of this compound
Metabolite | Pathway | Enzyme Involved |
---|---|---|
This compound | Prodrug | - |
This compound Sulfide | Active NSAID | Methionine sulfoxide reductase A |
This compound Sulfone | Oxidative metabolite | Cytochrome P450 |
Research indicates that this compound exerts its anticancer effects through several mechanisms:
- Induction of Apoptosis : this compound sensitizes cancer cells to oxidative stress, leading to increased production of reactive oxygen species (ROS) and subsequent apoptosis. This effect is particularly pronounced in lung cancer cells .
- Inhibition of Cyclooxygenases : The active metabolite SS selectively inhibits cyclooxygenase-1 (COX-1) and COX-2 enzymes, which play crucial roles in inflammation and tumorigenesis. This inhibition leads to decreased prostaglandin synthesis, which is associated with reduced tumor growth in various cancer models .
- Autophagic Apoptosis : Studies have shown that sulindac can induce autophagic apoptosis in specific neuronal populations, suggesting a broader impact on cellular survival pathways beyond cancer cells .
Case Studies and Clinical Findings
Several studies have highlighted the efficacy of this compound in cancer prevention and treatment:
- Familial Adenomatous Polyposis (FAP) : A randomized controlled trial demonstrated that patients with FAP treated with 300 mg/day of sulindac experienced significant regression in rectal polyps compared to placebo, with polyps decreasing in number by 56% and size by 65% over nine months .
- Breast Cancer Models : In triple-negative breast cancer models, SS inhibited mammosphere growth and demonstrated significant anti-tumor activity when combined with immune checkpoint inhibitors, highlighting its potential as an adjunct therapy in immunotherapy .
Table 2: Summary of Clinical Findings on this compound
Safety Profile and Adverse Effects
Despite its therapeutic benefits, the use of sulindac can be associated with hepatotoxicity and gastrointestinal side effects. Reports indicate cases of liver injury linked to sulindac treatment, necessitating careful monitoring during therapy .
Propriétés
IUPAC Name |
2-[(3Z)-6-fluoro-2-methyl-3-[[4-[(S)-methylsulfinyl]phenyl]methylidene]inden-1-yl]acetic acid | |
---|---|---|
Source | PubChem | |
URL | https://pubchem.ncbi.nlm.nih.gov | |
Description | Data deposited in or computed by PubChem | |
InChI |
InChI=1S/C20H17FO3S/c1-12-17(9-13-3-6-15(7-4-13)25(2)24)16-8-5-14(21)10-19(16)18(12)11-20(22)23/h3-10H,11H2,1-2H3,(H,22,23)/b17-9-/t25-/m0/s1 | |
Source | PubChem | |
URL | https://pubchem.ncbi.nlm.nih.gov | |
Description | Data deposited in or computed by PubChem | |
InChI Key |
MLKXDPUZXIRXEP-WXKFDZDZSA-N | |
Source | PubChem | |
URL | https://pubchem.ncbi.nlm.nih.gov | |
Description | Data deposited in or computed by PubChem | |
Canonical SMILES |
CC1=C(C2=C(C1=CC3=CC=C(C=C3)S(=O)C)C=CC(=C2)F)CC(=O)O | |
Source | PubChem | |
URL | https://pubchem.ncbi.nlm.nih.gov | |
Description | Data deposited in or computed by PubChem | |
Isomeric SMILES |
CC\1=C(C2=C(/C1=C\C3=CC=C(C=C3)[S@@](=O)C)C=CC(=C2)F)CC(=O)O | |
Source | PubChem | |
URL | https://pubchem.ncbi.nlm.nih.gov | |
Description | Data deposited in or computed by PubChem | |
Molecular Formula |
C20H17FO3S | |
Source | PubChem | |
URL | https://pubchem.ncbi.nlm.nih.gov | |
Description | Data deposited in or computed by PubChem | |
Molecular Weight |
356.4 g/mol | |
Source | PubChem | |
URL | https://pubchem.ncbi.nlm.nih.gov | |
Description | Data deposited in or computed by PubChem | |
CAS No. |
149116-77-8 | |
Record name | Sulindac, (S)- | |
Source | ChemIDplus | |
URL | https://pubchem.ncbi.nlm.nih.gov/substance/?source=chemidplus&sourceid=0149116778 | |
Description | ChemIDplus is a free, web search system that provides access to the structure and nomenclature authority files used for the identification of chemical substances cited in National Library of Medicine (NLM) databases, including the TOXNET system. | |
Record name | SULINDAC, (S)- | |
Source | FDA Global Substance Registration System (GSRS) | |
URL | https://gsrs.ncats.nih.gov/ginas/app/beta/substances/P969C405OM | |
Description | The FDA Global Substance Registration System (GSRS) enables the efficient and accurate exchange of information on what substances are in regulated products. Instead of relying on names, which vary across regulatory domains, countries, and regions, the GSRS knowledge base makes it possible for substances to be defined by standardized, scientific descriptions. | |
Explanation | Unless otherwise noted, the contents of the FDA website (www.fda.gov), both text and graphics, are not copyrighted. They are in the public domain and may be republished, reprinted and otherwise used freely by anyone without the need to obtain permission from FDA. Credit to the U.S. Food and Drug Administration as the source is appreciated but not required. | |
Synthesis routes and methods I
Procedure details
Synthesis routes and methods II
Procedure details
Retrosynthesis Analysis
AI-Powered Synthesis Planning: Our tool employs the Template_relevance Pistachio, Template_relevance Bkms_metabolic, Template_relevance Pistachio_ringbreaker, Template_relevance Reaxys, Template_relevance Reaxys_biocatalysis model, leveraging a vast database of chemical reactions to predict feasible synthetic routes.
One-Step Synthesis Focus: Specifically designed for one-step synthesis, it provides concise and direct routes for your target compounds, streamlining the synthesis process.
Accurate Predictions: Utilizing the extensive PISTACHIO, BKMS_METABOLIC, PISTACHIO_RINGBREAKER, REAXYS, REAXYS_BIOCATALYSIS database, our tool offers high-accuracy predictions, reflecting the latest in chemical research and data.
Strategy Settings
Precursor scoring | Relevance Heuristic |
---|---|
Min. plausibility | 0.01 |
Model | Template_relevance |
Template Set | Pistachio/Bkms_metabolic/Pistachio_ringbreaker/Reaxys/Reaxys_biocatalysis |
Top-N result to add to graph | 6 |
Feasible Synthetic Routes
Q1: What are the observed effects of (S)-Sulindac on normal and cancerous cells in the context of oxidative stress?
A2: Research suggests that both this compound and (R)-sulindac can protect normal lung cells from oxidative damage []. Intriguingly, both enantiomers also exhibit the ability to enhance the death of lung cancer cells when exposed to oxidative stress []. This selective action against cancerous cells, while protecting normal cells, points towards a potential therapeutic window for this compound in cancer treatment.
Q2: How do novel dithiolethione-modified NSAIDs like S-sulindac function as potential chemopreventive agents?
A3: S-sulindac, a dithiolethione-modified NSAID, exhibits promising chemopreventive activity by influencing both carcinogen activation and detoxification mechanisms []. Specifically, S-sulindac inhibits the activity and expression of carcinogen-activating enzymes, including CYP1A1, CYP1B1, and CYP1A2, in human hepatoma HepG2 and human colonic adenocarcinoma LS180 cells []. This inhibition occurs through transcriptional regulation of the aryl hydrocarbon receptor (AhR) pathway, where S-sulindac blocks the binding of carcinogen-activated AhR to the xenobiotic responsive element, thereby suppressing CYP1A1 expression []. Moreover, S-sulindac induces the expression of several carcinogen detoxification enzymes associated with the glutathione cycle, further contributing to its chemopreventive potential []. These findings suggest that S-sulindac can modulate the balance between carcinogen activation and detoxification, highlighting its potential as a chemopreventive agent.
Avertissement et informations sur les produits de recherche in vitro
Veuillez noter que tous les articles et informations sur les produits présentés sur BenchChem sont destinés uniquement à des fins informatives. Les produits disponibles à l'achat sur BenchChem sont spécifiquement conçus pour des études in vitro, qui sont réalisées en dehors des organismes vivants. Les études in vitro, dérivées du terme latin "in verre", impliquent des expériences réalisées dans des environnements de laboratoire contrôlés à l'aide de cellules ou de tissus. Il est important de noter que ces produits ne sont pas classés comme médicaments et n'ont pas reçu l'approbation de la FDA pour la prévention, le traitement ou la guérison de toute condition médicale, affection ou maladie. Nous devons souligner que toute forme d'introduction corporelle de ces produits chez les humains ou les animaux est strictement interdite par la loi. Il est essentiel de respecter ces directives pour assurer la conformité aux normes légales et éthiques en matière de recherche et d'expérimentation.