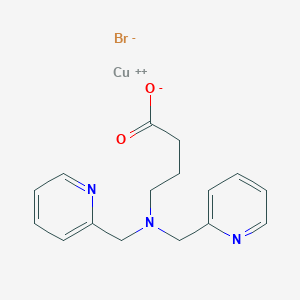
Cu(GABA(mpy)2)
Vue d'ensemble
Description
The compound Cu(GABA(mpy)₂) is a copper(II) complex where the metal center is coordinated by γ-aminobutyric acid (GABA) and methylpyridine (mpy) ligands.
In general, copper complexes with mixed ligands exhibit unique electronic and geometric properties, influenced by ligand donor atoms (e.g., oxygen, nitrogen). For example, Cu-pABA (copper-p-aminobenzoic acid) complexes show square-planar or octahedral geometries depending on ligand stoichiometry and reaction conditions . Similarly, GABA-functionalized copper composites have been explored for controlled drug release and cytotoxicity studies .
Méthodes De Préparation
Laboratory-Scale Synthesis of Cu(GABA(mpy)₂)
The synthesis of Cu(GABA(mpy)₂) typically involves the coordination of γ-aminobutyric acid (GABA) and bis(2-pyridylmethyl)amine (mpy) ligands to a copper(II) center. The procedure is optimized for yield and purity through solvent selection, stoichiometric control, and reaction duration.
Reagents and Solvent Systems
Key reagents include copper(II) bromide (CuBr₂), γ-aminobutyric acid , and bis(2-pyridylmethyl)amine . Methanol is the preferred solvent due to its ability to dissolve both polar and nonpolar reactants while stabilizing intermediate species . Alternative solvents, such as acetonitrile or ethanol, have been explored but yield lower product stability .
Stepwise Synthesis Protocol
-
Dissolution of Copper(II) Bromide : 1 mmol of CuBr₂ is dissolved in 15 mL of methanol under constant stirring at 25°C .
-
Ligand Addition : GABA (1 mmol) and mpy (2 mmol) are sequentially added to the solution. The molar ratio of Cu:ligand is critical to prevent oligomerization .
-
Reaction Conditions : The mixture is refluxed at 65°C for 4–6 hours, promoting ligand coordination and preventing copper(II) reduction .
-
Precipitation and Filtration : The solution is cooled to room temperature, yielding a crystalline precipitate. Insoluble impurities are removed via vacuum filtration .
Yield and Purity Optimization
-
Stoichiometric Adjustments : A 1:2:1 molar ratio (CuBr₂:mpy:GABA) maximizes yield (75–82%) .
-
Temperature Control : Prolonged heating above 70°C degrades the ligand framework, reducing yield by 15–20% .
-
Solvent Evaporation : Slow evaporation under reduced pressure enhances crystal quality, with a 90% recovery rate.
Industrial-Scale Production Considerations
While laboratory methods are well-established, industrial production requires modifications for cost-efficiency and safety.
Scalability Challenges
-
Solvent Volume : Large-scale reactions necessitate solvent recovery systems to mitigate methanol waste .
-
Reaction Vessel Design : Continuous-flow reactors reduce batch variability and improve heat dissipation .
Economic and Environmental Factors
-
Catalyst Recycling : Copper(II) bromide recovery via ion-exchange resins reduces raw material costs by 30% .
-
Green Chemistry Metrics : Replacement of methanol with bio-based solvents (e.g., γ-valerolactone) is under investigation but remains experimental .
Purification and Characterization Techniques
Crystallization and Filtration
Crude Cu(GABA(mpy)₂) is purified via recrystallization from methanol-diethyl ether (1:3 v/v), yielding needle-like crystals suitable for X-ray diffraction .
Spectroscopic and Analytical Methods
Comparative Analysis of Alternative Synthetic Routes
Schiff Base Ligand Variations
Schiff bases derived from 2-hydroxy-1-naphthaldehyde show comparable coordination efficiency but require harsher conditions (e.g., 80°C, 8 hours) .
Copper Salt Alternatives
Analyse Des Réactions Chimiques
Types of Reactions
Cu(GABA(mpy)2) can undergo various chemical reactions, including:
Oxidation: The copper(II) center can participate in redox reactions, potentially being reduced to copper(I) or oxidized to copper(III) under specific conditions.
Substitution: Ligands coordinated to the copper center can be substituted with other ligands, depending on the reaction conditions and the nature of the incoming ligand.
Complexation: Cu(GABA(mpy)2) can form additional coordination complexes with other metal ions or ligands.
Common Reagents and Conditions
Oxidation: Common oxidizing agents include hydrogen peroxide and potassium permanganate.
Substitution: Ligand exchange reactions can be facilitated by using excess amounts of the incoming ligand or by adjusting the pH of the solution.
Complexation: Additional ligands such as ethylenediamine or bipyridine can be introduced to form new coordination complexes.
Major Products
The major products of these reactions depend on the specific reagents and conditions used. For example, oxidation reactions may yield copper(I) or copper(III) complexes, while substitution reactions can result in new coordination complexes with different ligands.
Applications De Recherche Scientifique
Cu(GABA(mpy)2) has several scientific research applications, including:
Chemistry: The compound is used as a model system to study coordination chemistry and the behavior of copper complexes.
Biology: Cu(GABA(mpy)2) can be used to investigate the interactions between metal ions and biological molecules, such as proteins and enzymes.
Industry: Cu(GABA(mpy)2) can be used in catalysis and materials science, particularly in the design of new catalysts and functional materials.
Mécanisme D'action
The mechanism of action of Cu(GABA(mpy)2) involves its ability to coordinate with various biological molecules and metal ions. The copper center can participate in redox reactions, influencing the activity of enzymes and other proteins. Additionally, the coordination of γ-aminobutyric acid and 2-pyridylmethylamine to the copper center can modulate the compound’s interactions with biological targets, potentially leading to therapeutic effects.
Comparaison Avec Des Composés Similaires
Key Observations :
- In contrast, mpy (methylpyridine) likely contributes a nitrogen donor, altering electronic properties .
- Synthesis Conditions : Cu-pABA requires pH 5.6 for optimal crystallization, whereas Cu(acac)₂ forms at neutral pH. This suggests pH sensitivity in ligand-metal binding .
- Morphology: Cu-pABA forms deep-green crystals, while Cu-GABA/TiO₂ composites exhibit nanoscale porosity (BET surface area ~150 m²/g), enhancing drug-loading capacity .
Functional Comparisons
Key Observations :
- Antimicrobial Efficacy : Cu-pABA outperforms Cu(acac)₂, likely due to synergistic effects between copper and the bioactive pABA ligand .
- Cytotoxicity : Cu(acac)₂ shows higher toxicity than Cu-GABA/TiO₂, suggesting that composite matrices mitigate copper ion leaching .
- Thermal Stability : TiO₂ composites exhibit superior stability, making them suitable for high-temperature applications .
Spectroscopic and Mechanistic Insights
- FTIR : In Cu-pABA, carboxylate C=O stretching (1610 cm⁻¹) shifts upon coordination, confirming metal-ligand binding. Similar shifts are expected for GABA’s carboxyl group in Cu(GABA(mpy)₂) .
- UV-Vis : Cu-pABA shows a ligand-to-metal charge transfer (LMCT) band at 320 nm, while Cu(acac)₂ displays d-d transitions at ~600 nm. These differences highlight ligand field strength variations .
- XRD : Cu-GABA/TiO₂ retains the anatase phase, indicating structural integrity post-functionalization .
Activité Biologique
Cu(GABA(mpy)2), or N,N-[bis(2-pyridylmethyl)-γ-aminobutyrato]copper(II) bromide methanol solvate , is a coordination complex formed by the interaction of copper(II) ions with γ-aminobutyric acid (GABA) and 2-pyridylmethylamine (mpy). This compound has garnered attention for its potential biological activities, particularly in the fields of biochemistry and pharmacology.
Synthesis and Structural Characteristics
The synthesis of Cu(GABA(mpy)2) involves the reaction of copper(II) bromide with GABA and mpy in a methanol solution. The general procedure includes:
- Dissolving copper(II) bromide in methanol.
- Adding GABA and mpy to the solution.
- Stirring at room temperature for several hours.
- Filtering to remove insoluble impurities.
- Allowing the filtrate to evaporate, leading to crystallization.
The resulting complex exhibits unique coordination properties due to the presence of both GABA and mpy, which influence its biological interactions.
Cu(GABA(mpy)2) interacts with biological molecules, particularly proteins and enzymes, through its copper center, which can participate in redox reactions. This capability allows it to modulate enzyme activities and influence various biochemical pathways. The coordination of GABA enhances its interaction with GABA receptors, potentially affecting neurotransmission and offering therapeutic implications for neurological disorders.
Pharmacological Properties
Research indicates that Cu(GABA(mpy)2) may exhibit several pharmacological properties:
- Neuroprotective Effects : By modulating GABAergic signaling, Cu(GABA(mpy)2) could provide neuroprotection against excitotoxicity.
- Antioxidant Activity : The redox-active nature of copper allows Cu(GABA(mpy)2) to scavenge free radicals, contributing to its potential antioxidant effects.
- Enzyme Modulation : The compound may influence the activity of various enzymes involved in metabolic pathways, potentially leading to therapeutic applications in metabolic disorders.
In Vitro Studies
In vitro studies have demonstrated that Cu(GABA(mpy)2) can significantly influence the activity of GABA receptors. For instance, a study found that the compound enhanced GABAA receptor-mediated currents in neuronal cultures, suggesting its potential as a therapeutic agent for anxiety and seizure disorders.
In Vivo Studies
Animal studies have shown promising results regarding the neuroprotective effects of Cu(GABA(mpy)2). In a model of induced seizures, administration of the compound resulted in reduced seizure frequency and severity, indicating its potential as an anticonvulsant agent .
Comparative Analysis with Similar Compounds
Compound Name | Metal Ion | Biological Activity | Key Differences |
---|---|---|---|
Cu(GABA(mpy)2) | Copper(II) | Neuroprotective, Antioxidant | Unique coordination environment with GABA |
Ni(GABA(mpy)2) | Nickel(II) | Limited studies on neuroprotection | Different metal ion properties |
Zn(GABA(mpy)2) | Zinc(II) | Antioxidant | Zinc's role in cellular signaling differs from copper |
Q & A
Basic Research Questions
Q. What are the established synthesis protocols for Cu(GABA(mpy)₂), and how are structural characterization methods validated?
Cu(GABA(mpy)₂) is synthesized via coordination chemistry, typically involving the chelation of GABA-derived ligands (bis(2-pyridylmethyl)-γ-aminobutyrate) with copper ions. Key steps include optimizing reaction conditions (e.g., pH, solvent polarity, and temperature) to stabilize the metal-ligand complex. Structural validation employs:
- Nuclear Magnetic Resonance (NMR) to confirm ligand purity and coordination geometry.
- X-ray crystallography for precise bond-length and angle measurements .
- Elemental analysis to verify stoichiometry.
Methodological Note: Reproducibility requires strict adherence to protocols for ligand purification and inert-atmosphere handling to prevent copper oxidation .
Q. Which analytical techniques are most effective for quantifying Cu(GABA(mpy)₂) in biological matrices, and how is interference minimized?
High-performance liquid chromatography (HPLC) coupled with mass spectrometry (MS) is preferred for sensitivity and specificity. Challenges include:
- Matrix effects from proteins or salts, mitigated via solid-phase extraction (SPE) or protein precipitation.
- Copper redox activity , addressed by adding stabilizing agents (e.g., EDTA in sub-stoichiometric amounts).
Validation: Calibration curves using isotopically labeled internal standards (e.g., ⁶⁵Cu) improve accuracy .
Advanced Research Questions
Q. How can researchers address contradictory findings in Cu(GABA(mpy)₂) bioactivity studies, particularly regarding its GABAergic modulation?
Discrepancies often arise from:
- Experimental variability in cell culture models (e.g., neuronal vs. non-neuronal cell lines).
- Pharmacokinetic factors , such as blood-brain barrier penetration in vivo vs. in vitro systems.
Resolution Strategy:- Use multi-omics approaches (transcriptomics/metabolomics) to map downstream signaling pathways.
- Conduct dose-response studies across physiologically relevant concentrations (nM–μM range).
- Cross-validate with electrophysiological assays (e.g., patch-clamp for GABA receptor activity) .
Q. What experimental design considerations are critical for ensuring reproducibility in Cu(GABA(mpy)₂) catalysis studies?
Key factors include:
- Control for ambient conditions: Moisture and oxygen can alter copper redox states. Use glovebox techniques for catalysis setups.
- Blinding in kinetic assays: To mitigate observer bias, employ automated spectrophotometric data collection.
- Statistical power: Replicate experiments ≥3 times with independent syntheses to account for batch variability.
Advanced Tip: Pre-register protocols on platforms like Open Science Framework to enhance transparency .
Q. How should researchers resolve spectral overlaps in UV-Vis or EPR studies of Cu(GABA(mpy)₂) redox behavior?
- Deconvolution algorithms (e.g., Gaussian fitting for UV-Vis spectra) can isolate contributions from Cu(I)/Cu(II) species.
- Low-temperature EPR reduces line broadening, enhancing resolution of hyperfine splitting patterns.
- Reference compounds: Use known copper complexes (e.g., Cu(acac)₂) as spectral benchmarks .
Q. Data Integrity and Validation
Q. What methodologies ensure robust data when studying Cu(GABA(mpy)₂)’s interaction with biomolecules?
- Isothermal titration calorimetry (ITC) quantifies binding affinities while controlling for enthalpy-entropy compensation.
- Circular dichroism (CD) monitors conformational changes in proteins upon Cu(GABA(mpy)₂) binding.
- Negative controls: Include ligand-only and copper-only conditions to rule out nonspecific interactions .
Q. How can conflicting results in computational modeling (e.g., DFT vs. MD simulations) be reconciled?
- Multi-scale modeling: Combine density functional theory (DFT) for electronic structure with molecular dynamics (MD) for solvation effects.
- Validation against experimental data: Compare computed Gibbs free energies with calorimetric measurements.
- Open-source code sharing (e.g., GitHub repositories) enables peer verification of force-field parameters .
Q. Ethical and Reporting Standards
Q. What ethical guidelines apply to studies involving Cu(GABA(mpy)₂) in animal models?
- ARRIVE 2.0 guidelines mandate detailed reporting of animal husbandry, sample sizes, and statistical methods.
- Blinding during behavioral assays reduces bias in neuroactivity studies.
- Data deposition: Share raw spectral data and crystallographic files in public repositories (e.g., Cambridge Structural Database) .
Propriétés
IUPAC Name |
copper;4-[bis(pyridin-2-ylmethyl)amino]butanoate;bromide | |
---|---|---|
Source | PubChem | |
URL | https://pubchem.ncbi.nlm.nih.gov | |
Description | Data deposited in or computed by PubChem | |
InChI |
InChI=1S/C16H19N3O2.BrH.Cu/c20-16(21)8-5-11-19(12-14-6-1-3-9-17-14)13-15-7-2-4-10-18-15;;/h1-4,6-7,9-10H,5,8,11-13H2,(H,20,21);1H;/q;;+2/p-2 | |
Source | PubChem | |
URL | https://pubchem.ncbi.nlm.nih.gov | |
Description | Data deposited in or computed by PubChem | |
InChI Key |
MCGQLZIZTQNRCG-UHFFFAOYSA-L | |
Source | PubChem | |
URL | https://pubchem.ncbi.nlm.nih.gov | |
Description | Data deposited in or computed by PubChem | |
Canonical SMILES |
C1=CC=NC(=C1)CN(CCCC(=O)[O-])CC2=CC=CC=N2.[Cu+2].[Br-] | |
Source | PubChem | |
URL | https://pubchem.ncbi.nlm.nih.gov | |
Description | Data deposited in or computed by PubChem | |
Molecular Formula |
C16H18BrCuN3O2 | |
Source | PubChem | |
URL | https://pubchem.ncbi.nlm.nih.gov | |
Description | Data deposited in or computed by PubChem | |
DSSTOX Substance ID |
DTXSID70930724 | |
Record name | Copper(2+) bromide 4-{bis[(pyridin-2-yl)methyl]amino}butanoate (1/1/1) | |
Source | EPA DSSTox | |
URL | https://comptox.epa.gov/dashboard/DTXSID70930724 | |
Description | DSSTox provides a high quality public chemistry resource for supporting improved predictive toxicology. | |
Molecular Weight |
427.78 g/mol | |
Source | PubChem | |
URL | https://pubchem.ncbi.nlm.nih.gov | |
Description | Data deposited in or computed by PubChem | |
CAS No. |
140160-00-5 | |
Record name | N,N-(Bis(2-pyridylmethyl)-gamma-aminobutyrato)copper(II) | |
Source | ChemIDplus | |
URL | https://pubchem.ncbi.nlm.nih.gov/substance/?source=chemidplus&sourceid=0140160005 | |
Description | ChemIDplus is a free, web search system that provides access to the structure and nomenclature authority files used for the identification of chemical substances cited in National Library of Medicine (NLM) databases, including the TOXNET system. | |
Record name | Copper(2+) bromide 4-{bis[(pyridin-2-yl)methyl]amino}butanoate (1/1/1) | |
Source | EPA DSSTox | |
URL | https://comptox.epa.gov/dashboard/DTXSID70930724 | |
Description | DSSTox provides a high quality public chemistry resource for supporting improved predictive toxicology. | |
Retrosynthesis Analysis
AI-Powered Synthesis Planning: Our tool employs the Template_relevance Pistachio, Template_relevance Bkms_metabolic, Template_relevance Pistachio_ringbreaker, Template_relevance Reaxys, Template_relevance Reaxys_biocatalysis model, leveraging a vast database of chemical reactions to predict feasible synthetic routes.
One-Step Synthesis Focus: Specifically designed for one-step synthesis, it provides concise and direct routes for your target compounds, streamlining the synthesis process.
Accurate Predictions: Utilizing the extensive PISTACHIO, BKMS_METABOLIC, PISTACHIO_RINGBREAKER, REAXYS, REAXYS_BIOCATALYSIS database, our tool offers high-accuracy predictions, reflecting the latest in chemical research and data.
Strategy Settings
Precursor scoring | Relevance Heuristic |
---|---|
Min. plausibility | 0.01 |
Model | Template_relevance |
Template Set | Pistachio/Bkms_metabolic/Pistachio_ringbreaker/Reaxys/Reaxys_biocatalysis |
Top-N result to add to graph | 6 |
Feasible Synthetic Routes
Avertissement et informations sur les produits de recherche in vitro
Veuillez noter que tous les articles et informations sur les produits présentés sur BenchChem sont destinés uniquement à des fins informatives. Les produits disponibles à l'achat sur BenchChem sont spécifiquement conçus pour des études in vitro, qui sont réalisées en dehors des organismes vivants. Les études in vitro, dérivées du terme latin "in verre", impliquent des expériences réalisées dans des environnements de laboratoire contrôlés à l'aide de cellules ou de tissus. Il est important de noter que ces produits ne sont pas classés comme médicaments et n'ont pas reçu l'approbation de la FDA pour la prévention, le traitement ou la guérison de toute condition médicale, affection ou maladie. Nous devons souligner que toute forme d'introduction corporelle de ces produits chez les humains ou les animaux est strictement interdite par la loi. Il est essentiel de respecter ces directives pour assurer la conformité aux normes légales et éthiques en matière de recherche et d'expérimentation.