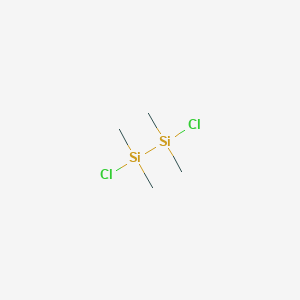
1,2-Dichlorotetraméthyldisilane
Vue d'ensemble
Description
1,2-Dichlorotetramethyldisilane is an organosilicon compound with the molecular formula C₄H₁₂Cl₂Si₂ and a molecular weight of 187.215 g/mol . It is also known by other names such as 1,2-dichloro-tetramethyldisilane . This compound is characterized by the presence of two silicon atoms bonded to each other, with each silicon atom also bonded to two methyl groups and one chlorine atom.
Applications De Recherche Scientifique
1,2-Dichlorotetramethyldisilane has several scientific research applications, including:
Mécanisme D'action
Target of Action
This compound is often used in the field of materials science and microelectronics , suggesting that its primary targets may be inorganic materials rather than biological entities.
Mode of Action
1,2-Dichlorotetramethyldisilane is known to undergo hydrolysis at low temperatures, resulting in the formation of cyclic and linear permethyl (oxadisilanes) . This suggests that the compound interacts with water molecules in its environment, leading to a change in its chemical structure .
Result of Action
Its use in materials science and microelectronics suggests that it may have significant effects on the physical properties of inorganic materials .
Action Environment
Environmental factors can influence the action, efficacy, and stability of 1,2-Dichlorotetramethyldisilane. For instance, the compound’s hydrolysis at low temperatures suggests that temperature is a key factor influencing its behavior . Additionally, the presence of water molecules in the environment is necessary for this hydrolysis reaction to occur .
Analyse Biochimique
Biochemical Properties
It is known to be a widely used organic silicon intermediate, often used as an organic silicon blocking agent in synthesis
Cellular Effects
It is known that it can cause severe skin burns and eye damage , suggesting that it may have significant cytotoxic effects
Molecular Mechanism
It is known to undergo hydrolysis to form 1,2-Dihydroxy-tetramethyldisilane
Temporal Effects in Laboratory Settings
It is known to be a flammable liquid with a flash point of 37 °C
Dosage Effects in Animal Models
Given its known cytotoxic effects , it is likely that high doses could have toxic or adverse effects
Metabolic Pathways
It is known to undergo hydrolysis to form 1,2-Dihydroxy-tetramethyldisilane
Transport and Distribution
Given its known cytotoxic effects , it is likely that it interacts with certain transporters or binding proteins
Subcellular Localization
Given its known cytotoxic effects , it is likely that it localizes to certain compartments or organelles
Méthodes De Préparation
1,2-Dichlorotetramethyldisilane can be synthesized through various methods. One common synthetic route involves the reaction of tetramethyldisilane with chlorine gas under controlled conditions . The reaction typically takes place at elevated temperatures and may require the presence of a catalyst to facilitate the chlorination process. Industrial production methods may involve similar reaction conditions but on a larger scale, with additional purification steps to ensure the desired product’s quality.
Analyse Des Réactions Chimiques
1,2-Dichlorotetramethyldisilane undergoes several types of chemical reactions, including:
Substitution Reactions: The chlorine atoms in the compound can be substituted with other functional groups through reactions with appropriate reagents.
Oxidation and Reduction Reactions: The compound can participate in oxidation and reduction reactions, although specific conditions and reagents for these reactions may vary.
Polymerization Reactions: It can be used as a precursor in the synthesis of various polymers, particularly in the preparation of silicon-based polymers.
Comparaison Avec Des Composés Similaires
1,2-Dichlorotetramethyldisilane can be compared with other similar compounds, such as:
Disilane, 1,2-dichloro-1,1,2,2-tetrakis(1-methylethyl)-: This compound has a similar structure but with different substituents on the silicon atoms.
1,1,2,2-Tetramethyl-1,2-bis(trimethylsilyloxy)disilane: Another related compound with different functional groups attached to the silicon atoms.
The uniqueness of disilane, 1,2-dichloro-1,1,2,2-tetramethyl- lies in its specific combination of chlorine and methyl groups, which imparts distinct reactivity and applications compared to its analogs.
Activité Biologique
1,2-Dichlorotetramethyldisilane (DCTMDS) is a silane compound known for its applications in various fields, including materials science and organic synthesis. Its unique structure allows for diverse chemical reactivity, particularly in the context of biological activity. This article provides a comprehensive overview of the biological activity of DCTMDS, including its mechanisms of action, potential therapeutic applications, and relevant case studies.
1,2-Dichlorotetramethyldisilane is characterized by the following properties:
- Molecular Formula : C4H12Cl2Si2
- Molecular Weight : 195.19 g/mol
- Boiling Point : 148-149 °C
- Density : 1.005 g/mL at 25 °C
- Hazard Classification : Flammable liquid, skin corrosive (GHS classification) .
Silylation Reactions
DCTMDS has been utilized in silylation reactions, which involve the introduction of silyl groups into organic molecules. This process enhances the stability and reactivity of various compounds, making them suitable for biological applications. For instance, DCTMDS has been used to synthesize biologically relevant compounds through silylation, contributing to drug development .
Inhibition Studies
Research indicates that DCTMDS may exhibit inhibitory effects on certain biological pathways. For example, studies have shown that derivatives synthesized from DCTMDS can act as inhibitors for specific enzymes and receptors involved in disease processes . This suggests potential therapeutic applications in treating conditions such as cancer and viral infections.
Study on Enzyme Inhibition
A notable study investigated the use of DCTMDS-derived compounds as inhibitors of acetylcholinesterase (AChE), an enzyme linked to neurodegenerative diseases. The synthesized analogs demonstrated varying degrees of inhibition, with some exhibiting IC50 values comparable to known AChE inhibitors . This finding highlights the potential of DCTMDS in developing neuroprotective agents.
Antiviral Activity
Another study explored the antiviral properties of compounds derived from DCTMDS against influenza A virus. The results indicated that certain derivatives effectively inhibited viral replication by interfering with the virus's ability to enter host cells . This positions DCTMDS as a candidate for further research in antiviral drug development.
Synthesis and Application in Drug Development
The synthesis of biologically active compounds using DCTMDS typically involves several steps:
- Silylation Reaction : DCTMDS is reacted with organic substrates under controlled conditions to introduce silyl groups.
- Purification : The resulting products are purified using standard techniques such as chromatography.
- Biological Testing : The purified compounds undergo biological testing to evaluate their activity against target enzymes or pathogens.
This method has been successfully applied in synthesizing various drug candidates, demonstrating DCTMDS's versatility in medicinal chemistry .
Propriétés
IUPAC Name |
chloro-[chloro(dimethyl)silyl]-dimethylsilane | |
---|---|---|
Source | PubChem | |
URL | https://pubchem.ncbi.nlm.nih.gov | |
Description | Data deposited in or computed by PubChem | |
InChI |
InChI=1S/C4H12Cl2Si2/c1-7(2,5)8(3,4)6/h1-4H3 | |
Source | PubChem | |
URL | https://pubchem.ncbi.nlm.nih.gov | |
Description | Data deposited in or computed by PubChem | |
InChI Key |
SFAZXBAPWCPIER-UHFFFAOYSA-N | |
Source | PubChem | |
URL | https://pubchem.ncbi.nlm.nih.gov | |
Description | Data deposited in or computed by PubChem | |
Canonical SMILES |
C[Si](C)([Si](C)(C)Cl)Cl | |
Source | PubChem | |
URL | https://pubchem.ncbi.nlm.nih.gov | |
Description | Data deposited in or computed by PubChem | |
Molecular Formula |
C4H12Cl2Si2 | |
Source | PubChem | |
URL | https://pubchem.ncbi.nlm.nih.gov | |
Description | Data deposited in or computed by PubChem | |
DSSTOX Substance ID |
DTXSID4040717 | |
Record name | 1,2-Dichloro-1,1,2,2-tetramethyldisilane | |
Source | EPA DSSTox | |
URL | https://comptox.epa.gov/dashboard/DTXSID4040717 | |
Description | DSSTox provides a high quality public chemistry resource for supporting improved predictive toxicology. | |
Molecular Weight |
187.21 g/mol | |
Source | PubChem | |
URL | https://pubchem.ncbi.nlm.nih.gov | |
Description | Data deposited in or computed by PubChem | |
CAS No. |
4342-61-4 | |
Record name | 1,2-Dichloro-1,1,2,2-tetramethyldisilane | |
Source | CAS Common Chemistry | |
URL | https://commonchemistry.cas.org/detail?cas_rn=4342-61-4 | |
Description | CAS Common Chemistry is an open community resource for accessing chemical information. Nearly 500,000 chemical substances from CAS REGISTRY cover areas of community interest, including common and frequently regulated chemicals, and those relevant to high school and undergraduate chemistry classes. This chemical information, curated by our expert scientists, is provided in alignment with our mission as a division of the American Chemical Society. | |
Explanation | The data from CAS Common Chemistry is provided under a CC-BY-NC 4.0 license, unless otherwise stated. | |
Record name | Disilane, 1,2-dichloro-1,1,2,2-tetramethyl- | |
Source | ChemIDplus | |
URL | https://pubchem.ncbi.nlm.nih.gov/substance/?source=chemidplus&sourceid=0004342614 | |
Description | ChemIDplus is a free, web search system that provides access to the structure and nomenclature authority files used for the identification of chemical substances cited in National Library of Medicine (NLM) databases, including the TOXNET system. | |
Record name | Disilane, 1,2-dichloro-1,1,2,2-tetramethyl- | |
Source | EPA Chemicals under the TSCA | |
URL | https://www.epa.gov/chemicals-under-tsca | |
Description | EPA Chemicals under the Toxic Substances Control Act (TSCA) collection contains information on chemicals and their regulations under TSCA, including non-confidential content from the TSCA Chemical Substance Inventory and Chemical Data Reporting. | |
Record name | 1,2-Dichloro-1,1,2,2-tetramethyldisilane | |
Source | EPA DSSTox | |
URL | https://comptox.epa.gov/dashboard/DTXSID4040717 | |
Description | DSSTox provides a high quality public chemistry resource for supporting improved predictive toxicology. | |
Record name | 1,2-dichloro-1,1,2,2-tetramethyldisilane | |
Source | European Chemicals Agency (ECHA) | |
URL | https://echa.europa.eu/substance-information/-/substanceinfo/100.022.183 | |
Description | The European Chemicals Agency (ECHA) is an agency of the European Union which is the driving force among regulatory authorities in implementing the EU's groundbreaking chemicals legislation for the benefit of human health and the environment as well as for innovation and competitiveness. | |
Explanation | Use of the information, documents and data from the ECHA website is subject to the terms and conditions of this Legal Notice, and subject to other binding limitations provided for under applicable law, the information, documents and data made available on the ECHA website may be reproduced, distributed and/or used, totally or in part, for non-commercial purposes provided that ECHA is acknowledged as the source: "Source: European Chemicals Agency, http://echa.europa.eu/". Such acknowledgement must be included in each copy of the material. ECHA permits and encourages organisations and individuals to create links to the ECHA website under the following cumulative conditions: Links can only be made to webpages that provide a link to the Legal Notice page. | |
Retrosynthesis Analysis
AI-Powered Synthesis Planning: Our tool employs the Template_relevance Pistachio, Template_relevance Bkms_metabolic, Template_relevance Pistachio_ringbreaker, Template_relevance Reaxys, Template_relevance Reaxys_biocatalysis model, leveraging a vast database of chemical reactions to predict feasible synthetic routes.
One-Step Synthesis Focus: Specifically designed for one-step synthesis, it provides concise and direct routes for your target compounds, streamlining the synthesis process.
Accurate Predictions: Utilizing the extensive PISTACHIO, BKMS_METABOLIC, PISTACHIO_RINGBREAKER, REAXYS, REAXYS_BIOCATALYSIS database, our tool offers high-accuracy predictions, reflecting the latest in chemical research and data.
Strategy Settings
Precursor scoring | Relevance Heuristic |
---|---|
Min. plausibility | 0.01 |
Model | Template_relevance |
Template Set | Pistachio/Bkms_metabolic/Pistachio_ringbreaker/Reaxys/Reaxys_biocatalysis |
Top-N result to add to graph | 6 |
Feasible Synthetic Routes
Q1: What is the molecular formula and weight of 1,2-dichlorotetramethyldisilane?
A1: Its molecular formula is Si2C4H12Cl2, and its molecular weight is 187.24 g/mol.
Q2: What spectroscopic data are available for characterizing 1,2-dichlorotetramethyldisilane?
A2: 1,2-Dichlorotetramethyldisilane has been characterized using various spectroscopic techniques, including:
- NMR Spectroscopy: 1H, 13C, 29Si NMR spectroscopy provides information about the structure and conformation of the molecule. For instance, 29Si NMR can distinguish between different isomers and conformers. [, ]
- Raman Spectroscopy: This technique can be used to study the rotational isomerism of the molecule and to determine the composition of different conformers in the gas phase. []
- Gas Electron Diffraction: This method provides information about the bond lengths and angles in the molecule, which can be used to determine its structure in the gas phase. [, ]
Q3: What is the stability of 1,2-dichlorotetramethyldisilane under different conditions?
A3: 1,2-Dichlorotetramethyldisilane is sensitive to moisture and should be handled under inert atmosphere. It can undergo hydrolysis, particularly at elevated temperatures, yielding various siloxane products. The composition and ratio of these hydrolysis products are temperature-dependent. []
Q4: How is 1,2-dichlorotetramethyldisilane used in the synthesis of cyclic compounds?
A4: 1,2-Dichlorotetramethyldisilane serves as a precursor for a variety of cyclic compounds, particularly heterocycles:
- Silacycles: It reacts with dilithiated compounds, such as 1,2-dilithio-1,2-dicarba-closo-dodecaborane(12), to form cyclic compounds containing silicon and other heteroatoms (e.g., tellurium, selenium). [, ]
- Disilacyclobutanes: Reacting a dilithiodisilane with 1,2-dichlorotetramethyldisilane yields four-membered cyclic disilacyclobutanes. []
- Diazasilacycloalkanes: Reacting 1,2-dichlorotetramethyldisilane with diamines like N,N'-dimethylethylenediamine or N,N'-diphenylethylenediamine yields six-membered diazasilacyclohexane derivatives. []
Q5: Can 1,2-dichlorotetramethyldisilane be used in palladium-catalyzed reactions?
A5: Yes, it acts as a reducing agent in palladium-catalyzed reductive coupling reactions. For instance, it couples with benzylidene dichlorides or benzylidyne trichlorides in the presence of a palladium catalyst to afford (E)-stilbenes or (E)- and (Z)-α,β-dichlorostilbenes, respectively. []
Q6: How is 1,2-dichlorotetramethyldisilane used in the synthesis of polymers?
A6: 1,2-Dichlorotetramethyldisilane is a valuable precursor for synthesizing disilane-containing polymers:
- Polyamides and Polyimides: Reacting diaminodisilanes, synthesized from 1,2-dichlorotetramethyldisilane, with bisphenols containing amide or imide groups produces disilane-containing polyamides and polyimides. These polymers exhibit good solubility in organic solvents and possess thermal stability up to 300°C. []
- Silaanthracenophanes: Silylation of 1,8-di(lithioethynyl)anthracene with 1,2-dichlorotetramethyldisilane yields silaanthracenophanes, which can be further polymerized. []
- Polymers with Metal Centers: 1,2-Dichlorotetramethyldisilane enables the synthesis of ansa-[n]silacyclopentadienyl-cycloheptatrienyl-chromium complexes, which can undergo ring-opening polymerization to yield polymers bearing chromium in the backbone. []
Q7: Can 1,2-dichlorotetramethyldisilane be used to introduce silicon bridges into molecules?
A7: Absolutely. It serves as a reagent for introducing tetramethyldisilylene bridges:
- Silafluorenes: Lithiation of 2,2',6,6'-tetrabromobiphenyls followed by silylation with 1,2-dichlorotetramethyldisilane affords 4,5-tetramethyldisilylene-bridged 9-silafluorenes. This modification significantly impacts the photophysical properties of the silafluorene, inducing red shifts in absorption and fluorescence spectra. []
- Dicyclopentadienyl Ligands: Reaction with a dilithio compound like Li2[H3C5(SiMe2)(Si2Me4)C5H3] allows the introduction of a tetramethyldisilanyl bridge between two cyclopentadienyl units, creating a novel double-bridged dicyclopentadiene ligand. This ligand can be complexed with various metals, such as Zr and Ru, to form unique organometallic compounds. []
Q8: Have there been computational studies on 1,2-dichlorotetramethyldisilane and its derivatives?
A8: Yes, computational studies have been employed to understand the conformational preferences and electronic structures of 1,2-dichlorotetramethyldisilane and its derivatives. For example:
- Conformational Analysis: Ab initio calculations, combined with gas electron diffraction data, have been used to determine the structure and composition of the rotational conformers of 1,2-dichlorotetramethyldisilane. [, ]
- Aryl-Aryl Interactions: Symmetry-adapted perturbation theory (SAPT) calculations have been used to analyze the contributions of different intermolecular forces, such as London dispersion interactions, to the overall stability of aryl-substituted 1,2-diaryldisilanes. []
- Electronic Structure: Density functional theory (DFT) calculations have been employed to study the electronic structure and properties of silicon-bridged silafluorenes, revealing the impact of the silicon bridge on the HOMO and LUMO levels. []
Avertissement et informations sur les produits de recherche in vitro
Veuillez noter que tous les articles et informations sur les produits présentés sur BenchChem sont destinés uniquement à des fins informatives. Les produits disponibles à l'achat sur BenchChem sont spécifiquement conçus pour des études in vitro, qui sont réalisées en dehors des organismes vivants. Les études in vitro, dérivées du terme latin "in verre", impliquent des expériences réalisées dans des environnements de laboratoire contrôlés à l'aide de cellules ou de tissus. Il est important de noter que ces produits ne sont pas classés comme médicaments et n'ont pas reçu l'approbation de la FDA pour la prévention, le traitement ou la guérison de toute condition médicale, affection ou maladie. Nous devons souligner que toute forme d'introduction corporelle de ces produits chez les humains ou les animaux est strictement interdite par la loi. Il est essentiel de respecter ces directives pour assurer la conformité aux normes légales et éthiques en matière de recherche et d'expérimentation.