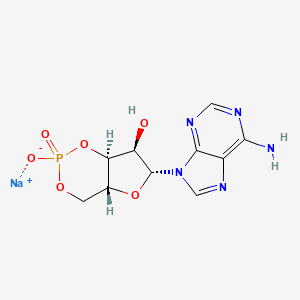
Cyclic AMP sodium
Vue d'ensemble
Description
Cyclic adenosine monophosphate sodium, commonly known as cyclic AMP sodium, is a derivative of adenosine triphosphate. It plays a crucial role as a second messenger in various biological processes, including the regulation of glycogen, sugar, and lipid metabolism. This compound is involved in the activation of protein kinases and the regulation of ion channel conductance.
Méthodes De Préparation
Synthetic Routes and Reaction Conditions
Cyclic AMP sodium is synthesized from adenosine triphosphate through the action of the enzyme adenylate cyclase. The reaction involves the conversion of adenosine triphosphate to cyclic AMP, which is then neutralized with sodium hydroxide to form this compound. The reaction conditions typically require a buffered aqueous solution and a controlled temperature to ensure the stability of the compound.
Industrial Production Methods
Industrial production of this compound involves large-scale fermentation processes using genetically engineered microorganisms that overexpress adenylate cyclase. The fermentation broth is then subjected to a series of purification steps, including filtration, ion exchange chromatography, and crystallization, to obtain high-purity this compound.
Analyse Des Réactions Chimiques
Hydrolysis by Phosphodiesterases
Cyclic AMP sodium undergoes enzymatic hydrolysis via phosphodiesterases (PDEs), which cleave the cyclic phosphate bond to yield adenosine monophosphate (AMP). This reaction regulates intracellular cAMP levels and downstream signaling.
Key Findings:
- Enzyme specificity : PDEs hydrolyze cAMP with a of 2–10 μM, depending on isoform specificity .
- Reaction rate : Catalyzed hydrolysis occurs rapidly (seconds to minutes) under physiological conditions (37°C, pH 7.4) .
- Inhibition : Methylxanthines (e.g., theophylline) and selective PDE inhibitors (e.g., rolipram) reduce hydrolysis rates by >90% .
Table 1: Hydrolysis Parameters of cAMP Sodium
Parameter | Value | Conditions | Source |
---|---|---|---|
(PDE4) | 4.2 μM | 37°C, pH 7.4 | |
12 nmol/min/mg protein | 37°C, pH 7.4 | ||
Half-life (in vivo) | 10–30 minutes | Mammalian cells |
Non-Enzymatic Oligomerization
Under dehydrating conditions, cAMP sodium forms short RNA-like oligomers via non-enzymatic transphosphorylation. This reaction is relevant to prebiotic chemistry and RNA evolution .
Reaction Conditions:
Key Findings:
- Efficiency : Oligomerization of cAMP is less efficient than cGMP due to unfavorable in-line attack angles in crystal packing (Table 2) .
- Product length : Yields dimers to pentamers, compared to cGMP’s heptamers under identical conditions .
Table 2: Oligomerization Comparison of cAMP vs. cGMP
Parameter | cAMP Sodium | cGMP Sodium |
---|---|---|
Optimal temperature | 80°C | 30°C |
Average product length | 3–5 nucleotides | 5–7 nucleotides |
Reaction time | 2–4 weeks | 1–2 weeks |
Stability and Degradation Under Buffer Conditions
This compound exhibits pH-dependent stability, with degradation accelerated in acidic or highly alkaline conditions.
Key Findings:
- pH stability : Stable at pH 6.0–8.0 (25°C); degradation increases by 50% at pH <5.0 or >9.0 .
- Thermal stability : Decomposes at >100°C, forming adenine and inorganic phosphate .
Table 3: Stability of cAMP Sodium in Buffer Solutions
pH | Degradation Rate (25°C) | Major Degradation Products |
---|---|---|
5.0 | 15%/week | AMP, adenine |
7.4 | <2%/week | None |
9.0 | 20%/week | AMP, inorganic phosphate |
Interaction with Metal Ions
This compound forms coordination complexes with divalent cations (e.g., Mg²⁺, Ca²⁺), modulating its biological activity .
Key Findings:
Applications De Recherche Scientifique
Neuroscience Applications
Cyclic AMP plays a significant role in neural signaling and development. Research indicates that sodium cyclic AMP is involved in the modulation of taste responses in rats, particularly regarding sodium chloride (NaCl) perception.
- Case Study : A study investigated the effect of sodium cyclic AMP on taste receptor cells in young rats. The application of a membrane-permeable form of cyclic AMP (8-chlorophenylthio-cAMP) enhanced NaCl responses significantly, demonstrating that cyclic AMP regulates sodium taste sensitivity during postnatal development. This effect was correlated with increased expression and trafficking of epithelial sodium channels (ENaCs) in taste receptor cells .
Renal Physiology
Sodium cyclic AMP is essential in regulating renal function and sodium reabsorption, particularly in conditions such as cirrhosis.
- Case Study : A study focused on the role of cyclic AMP in renal dysfunction associated with cirrhosis. It was found that administration of sodium cyclic AMP significantly increased sodium and phosphate excretion in control rats but had limited effects in cirrhotic rats with ascites due to increased phosphodiesterase activity. This suggests that enhancing cyclic AMP signaling could be a therapeutic target for managing renal complications in liver disease .
Cellular Biology
In cellular biology, sodium cyclic AMP is recognized for its role in regulating cell growth, differentiation, and apoptosis.
- Mechanism of Action : Cyclic AMP influences various cellular processes by activating protein kinase A (PKA), which phosphorylates specific target proteins leading to altered cellular functions. For instance, it has been shown to increase the surface expression of Na,K-ATPase in renal cells, thereby enhancing sodium transport .
- Case Study : Research demonstrated that cyclic AMP stimulates Na,K-ATPase activity by translocating active pump units to the plasma membrane, which is crucial for maintaining electrolyte balance and cellular homeostasis .
Therapeutic Applications
Sodium cyclic AMP has been targeted for therapeutic interventions across several diseases.
- Chronic Obstructive Pulmonary Disease (COPD) : Cyclic AMP signaling pathways are implicated in the pathogenesis of COPD. Studies indicate that manipulating these pathways may offer new therapeutic strategies for managing airway inflammation and remodeling associated with this disease .
- Cancer Research : Cyclic AMP has been studied for its potential role in cancer therapy, particularly its ability to regulate cell proliferation and apoptosis. For example, it has been shown to restore controlled growth in transformed cells, indicating its regulatory effects on tumor biology .
Data Tables
Mécanisme D'action
Cyclic AMP sodium exerts its effects by activating protein kinase A, which then phosphorylates various target proteins, leading to changes in their activity. This activation is mediated through the binding of cyclic AMP to the regulatory subunits of protein kinase A, causing a conformational change that releases the catalytic subunits. These catalytic subunits then phosphorylate specific serine or threonine residues on target proteins, modulating their function. The pathways involved include the regulation of glycogen metabolism, lipid metabolism, and ion channel conductance.
Comparaison Avec Des Composés Similaires
Similar Compounds
Cyclic guanosine monophosphate sodium: Another second messenger involved in signal transduction.
Adenosine monophosphate sodium: A nucleotide involved in energy metabolism.
Adenosine triphosphate sodium: The primary energy carrier in cells.
Uniqueness
Cyclic AMP sodium is unique in its ability to act as a second messenger that regulates a wide range of physiological processes. Unlike cyclic guanosine monophosphate sodium, which primarily mediates nitric oxide signaling, this compound is involved in a broader spectrum of cellular responses. Its role in activating protein kinase A and regulating metabolic pathways distinguishes it from other nucleotides like adenosine monophosphate sodium and adenosine triphosphate sodium.
Activité Biologique
Cyclic adenosine monophosphate (cAMP) is a crucial second messenger in various biological processes, influencing cellular signaling pathways that regulate physiological functions. The sodium salt of cAMP, commonly referred to as cyclic AMP sodium, is utilized in research to study its biological activity and therapeutic potential. This article delves into the biological activity of this compound, highlighting its mechanisms, effects on specific tissues, and its implications in various physiological and pathological conditions.
Cyclic AMP exerts its effects primarily through the activation of protein kinase A (PKA), which phosphorylates target proteins, leading to altered cellular responses. Additionally, cAMP can modulate ion channels and other signaling pathways, influencing cell function.
Key Mechanisms:
- Activation of Protein Kinase A (PKA) : cAMP binds to the regulatory subunits of PKA, causing a conformational change that releases the catalytic subunits, which then phosphorylate serine and threonine residues on target proteins.
- Regulation of Ion Channels : cAMP can influence the activity of cyclic-nucleotide-gated ion channels, affecting calcium and sodium ion flux across cell membranes, which is critical for excitability in neurons and muscle cells .
- Modulation of Na,K-ATPase Activity : Studies indicate that cAMP increases the activity and surface expression of Na,K-ATPase in renal cells, enhancing sodium transport .
Biological Effects
The biological effects of this compound are diverse and tissue-specific. Here are some notable findings:
Renal Function
This compound plays a significant role in renal physiology by regulating sodium reabsorption. It has been shown to:
- Increase Na,K-ATPase activity in renal cortical collecting ducts (CCDs), enhancing sodium transport across the cell membrane .
- Influence adenylyl cyclase activity, which is critical for cAMP synthesis in response to various hormonal signals.
Cardiovascular Effects
In cardiovascular tissues, cAMP influences:
- Vascular smooth muscle relaxation through modulation of calcium levels and enhancement of nitric oxide production .
- Cardiac myocyte contractility by increasing intracellular calcium levels via PKA-mediated phosphorylation .
Case Studies
Several studies have explored the effects of this compound on specific physiological processes:
- Study on Renal Cells : A study demonstrated that db-cAMP (a stable analog of cAMP) increased Na,K-ATPase activity by 2-fold in rat CCDs. This effect was associated with enhanced surface expression of the enzyme, indicating a mechanism for increased sodium reabsorption .
- Impact on Vascular Function : Research indicated that cyclic AMP could enhance the relaxant effects of vasodilators like papaverine by increasing intracellular cAMP levels, leading to improved vascular function under pathological conditions such as hypertension .
- Sodium Loading and Depletion : A study evaluated how sodium loading affected cAMP levels in rat aorta. Results showed that increased sodium intake led to elevated cAMP concentrations, suggesting a feedback mechanism where sodium balance influences cyclic nucleotide levels .
Data Table: Summary of Biological Effects
Biological Activity | Effect | Tissue/Cell Type |
---|---|---|
Na,K-ATPase Activation | Increased sodium transport | Renal Cortical Collecting Ducts |
Vascular Relaxation | Enhanced vasodilation | Vascular Smooth Muscle |
Cardiac Contractility | Increased force of contraction | Cardiac Myocytes |
Modulation of Ion Channels | Altered calcium and sodium flux | Neurons and Muscle Cells |
Q & A
Basic Research Questions
Q. How can researchers quantify intracellular cyclic AMP (cAMP) levels in different cell types, and what methodological considerations are critical for accuracy?
- Methodology :
- Radioimmunoassay (RIA) : Use acidified cell lysates (e.g., 3 M HClO₄) to stabilize cAMP, followed by neutralization with freon/amine. Quantify cAMP using a commercial RIA kit (e.g., [¹²⁵I]-cAMP), ensuring phosphodiesterase inhibitors (e.g., IBMX) are included during cell lysis to prevent cAMP degradation .
- Enzyme Immunoassay (EIA) : Neutralize lysates post-centrifugation (15,000×g) and use competitive binding assays (e.g., Amersham-Biotrak system) with cAMP-specific antibodies. Normalize data to total protein content via bicinchoninic acid (BCA) assays .
- Critical Considerations :
- Sample Handling : Rapid termination of reactions (e.g., HCl addition) and cold centrifugation (4°C) to preserve cAMP integrity .
- Phosphodiesterase Inhibition : Use IBMX (100–500 μM) to block cAMP hydrolysis during experiments .
Q. What experimental approaches distinguish cAMP from its structural analog AMP in biochemical assays?
- Structural Analysis :
- Cyclic vs. Linear Phosphate : cAMP forms a 3’,5’-cyclic phosphate ring, detectable via NMR or mass spectrometry. AMP lacks this cyclic structure, appearing as 5’-adenylic acid in chromatographic separations .
- Functional Assays :
- Protein Kinase A (PKA) Activation : cAMP (not AMP) binds PKA’s regulatory subunits, releasing catalytic subunits. Measure PKA activity via phosphorylation of kemptide substrates or [³²P]-ATP incorporation .
Q. How do researchers isolate cAMP-dependent signaling effects from other secondary messengers in complex cellular systems?
- Pharmacological Tools :
- Adenylyl Cyclase Activators : Use forskolin (10–50 μM) or receptor-specific agonists (e.g., β₂-adrenoceptor agonist clenbuterol) to selectively elevate cAMP .
- PKA Inhibitors : Apply H-89 (1–10 μM) or Rp-cAMPS to block PKA-mediated effects .
- Genetic Approaches :
- CRISPR/Cas9 knockout of cAMP effectors (e.g., EPAC or PKA subunits) to dissect pathway-specific roles .
Advanced Research Questions
Q. What mechanisms explain contradictory findings in cAMP’s role in NF-κB regulation, and how can these be resolved experimentally?
- Context-Dependent Signaling :
- Anti-Inflammatory vs. Pro-Inflammatory Effects : In immune cells, cAMP/PKA inhibits NF-κB by competing for coactivators (e.g., CBP/p300). However, in endothelial cells, cAMP may enhance NF-κB via CREB phosphorylation, depending on CBP availability .
- Resolution Strategies :
- Cell-Type-Specific Profiling : Compare cAMP responses in primary monocytes vs. endothelial cells using ChIP-seq to map CBP/p65 binding dynamics .
- Kinetic Studies : Time-course experiments (e.g., 2–60 min post-stimulation) to capture transient vs. sustained cAMP effects .
Q. How do compartmentalized cAMP pools influence divergent signaling outcomes, and what tools validate spatial resolution in live cells?
- Subcellular Microdomains :
- FRET-Based Sensors : Use Epac1-cAMP or AKAR3 reporters to visualize cAMP gradients in organelles (e.g., mitochondria vs. nucleus) .
- Pharmacological Probes :
- Membrane-Permeable Analogs : Apply 8-Br-cAMP (non-compartmentalized) vs. db-cAMP (compartment-restricted) to test localized effects .
Q. What novel mechanisms of cAMP signaling challenge the classical PKA/EPAC dichotomy, and how are these being investigated?
- Emerging Pathways :
- Cross-Talk with PI3K/PKB : cAMP via EPAC activates PI3K-dependent PKB, while PKA inhibits PKB. Use Epac-specific agonists (e.g., 8-pCPT-2’-O-Me-cAMP) and PKA inhibitors to dissect opposing effects .
- Non-Canonical Targets : cAMP-gated ion channels (CNGs) in neuronal cells modulate calcium influx independently of PKA/EPAC. Patch-clamp electrophysiology combined with cAMP uncaging (e.g., NPE-caged cAMP) can isolate these effects .
Q. How do researchers reconcile discrepancies in cAMP-mediated gene regulation across prokaryotic vs. eukaryotic systems?
- Prokaryotic Models :
- CRP-cAMP Complex : In E. coli, cAMP binds catabolite activator protein (CRP) to activate catabolic operons. Use EMSA or lacZ reporter assays to study promoter binding .
- Eukaryotic Systems :
- Chromatin Remodeling : cAMP/PKA phosphorylates histones (e.g., H3S10) to regulate accessibility. Compare ChIP-seq data from bacterial and mammalian cells to identify conserved vs. divergent mechanisms .
Q. Methodological Resources
- Key Protocols :
- cAMP Extraction : Acidified ethanol lysis (0.1 M HCl) for tissue samples .
- Kinase Activity Assays : Measure PKA activity ratios (radioactivity without/with cAMP) using phosphocellulose paper binding .
- Data Analysis :
- Use asymmetric sigmoid curve-fitting (e.g., Fig.P software) for dose-response studies and EC₅₀/Emax calculation .
Propriétés
Numéro CAS |
37839-81-9 |
---|---|
Formule moléculaire |
C10H12N5NaO6P |
Poids moléculaire |
352.20 g/mol |
Nom IUPAC |
sodium;(4aR,6R,7R,7aS)-6-(6-aminopurin-9-yl)-2-oxido-2-oxo-4a,6,7,7a-tetrahydro-4H-furo[3,2-d][1,3,2]dioxaphosphinin-7-ol |
InChI |
InChI=1S/C10H12N5O6P.Na/c11-8-5-9(13-2-12-8)15(3-14-5)10-6(16)7-4(20-10)1-19-22(17,18)21-7;/h2-4,6-7,10,16H,1H2,(H,17,18)(H2,11,12,13);/t4-,6-,7-,10-;/m1./s1 |
Clé InChI |
QJOMQLIYJMMGDA-MCDZGGTQSA-N |
SMILES |
C1C2C(C(C(O2)N3C=NC4=C(N=CN=C43)N)O)OP(=O)(O1)[O-].[Na+] |
SMILES isomérique |
C1[C@@H]2[C@H]([C@H]([C@@H](O2)N3C=NC4=C(N=CN=C43)N)O)OP(=O)(O1)O.[Na] |
SMILES canonique |
C1C2C(C(C(O2)N3C=NC4=C(N=CN=C43)N)O)OP(=O)(O1)O.[Na] |
Numéros CAS associés |
60-92-4 (Parent) |
Origine du produit |
United States |
Retrosynthesis Analysis
AI-Powered Synthesis Planning: Our tool employs the Template_relevance Pistachio, Template_relevance Bkms_metabolic, Template_relevance Pistachio_ringbreaker, Template_relevance Reaxys, Template_relevance Reaxys_biocatalysis model, leveraging a vast database of chemical reactions to predict feasible synthetic routes.
One-Step Synthesis Focus: Specifically designed for one-step synthesis, it provides concise and direct routes for your target compounds, streamlining the synthesis process.
Accurate Predictions: Utilizing the extensive PISTACHIO, BKMS_METABOLIC, PISTACHIO_RINGBREAKER, REAXYS, REAXYS_BIOCATALYSIS database, our tool offers high-accuracy predictions, reflecting the latest in chemical research and data.
Strategy Settings
Precursor scoring | Relevance Heuristic |
---|---|
Min. plausibility | 0.01 |
Model | Template_relevance |
Template Set | Pistachio/Bkms_metabolic/Pistachio_ringbreaker/Reaxys/Reaxys_biocatalysis |
Top-N result to add to graph | 6 |
Feasible Synthetic Routes
Avertissement et informations sur les produits de recherche in vitro
Veuillez noter que tous les articles et informations sur les produits présentés sur BenchChem sont destinés uniquement à des fins informatives. Les produits disponibles à l'achat sur BenchChem sont spécifiquement conçus pour des études in vitro, qui sont réalisées en dehors des organismes vivants. Les études in vitro, dérivées du terme latin "in verre", impliquent des expériences réalisées dans des environnements de laboratoire contrôlés à l'aide de cellules ou de tissus. Il est important de noter que ces produits ne sont pas classés comme médicaments et n'ont pas reçu l'approbation de la FDA pour la prévention, le traitement ou la guérison de toute condition médicale, affection ou maladie. Nous devons souligner que toute forme d'introduction corporelle de ces produits chez les humains ou les animaux est strictement interdite par la loi. Il est essentiel de respecter ces directives pour assurer la conformité aux normes légales et éthiques en matière de recherche et d'expérimentation.